Freezing and refrigeration (i.e., cold storage) are among the oldest methods of food preservation, but it was not until 1875 that a mechanical ammonia refrigeration system capable of supporting commercial refrigerated warehousing and freezing was invested. This major advance was hampered by the lack of proper facilities, a prime requirement for any refrigerated or frozen food industry. Thus, as late as the 1920s, food delivered to a market in a frozen state commonly thawed before it could be brought home or else thawed in household ice boxes and generally was of marginal to poor quality. Starting in the 1920s, Clarence Birdseye pioneered research on quick-freezing processes, equipment, frozen products, and frozen food packaging. As household refrigerators and freezers became more common, the modern frozen food industry grew rapidly.
Refrigeration today markedly influences the practices of agriculture and marketing and sets the economic climate of the food industry. Without mechanical refrigeration in transit, much of world trade in perishable food commodities would be impossible. Large cities that are distant from growing areas would cease to enjoy abundant fruits and vegetables. Refrigeration and cold storage equalize food prices throughout the year and make products available year round. Without them, prices would be very low at time of harvest and extremely high later on, if indeed the foods were available at all.
DISTINCTION BETWEEN REFRIGERATION AND FREEZING
The difference between refrigeration and cool storage on the one hand and freezing and frozen storage on the other should be noted. Cool storage generally refers to storage at temperatures above freezing, from about 16 C down to -2C. Commercial and household refrigerators are usually operated at 4.5-7C. Commercial refrigerators sometimes are operated at a slightly lower temperature when a particular food is being favored. Whereas pure water will freeze at 0 C, most foods will not begin to freeze until about -2C or lower. Frozen storage refers to storage at temperatures that maintain food in frozen condition. Good frozen storage generally requires temperatures of -18C or below. Refrigerated or cool storage generally will preserve perishable food for days or weeks, depending on the food. Frozen storage will preserve foods for months or even years if properly packaged.
Further distinctions between refrigeration and freezing temperatures are related to microorganism activity (Fig. 9.1). Most food spoilage microorganisms grow rapidly at temperatures above 10 C but some grow at temperatures below 0 C as long as there is unfrozen water available.
Food held under good refrigeration temperatures can still spoil due to microorganism growth. However, until recently, it was thought that although properly refrigerated food could spoil due to undesirable changes in odor, flavor, and appearance, this did not cause a safety problem because disease-causing organisms did not grow appreciably at these low temperatures. Indeed, this is true for many such microorganisms, but in recent years, food scientists have found that some food-poisoning microorganisms grow, albeit slowly, at temperatures as low as 3.3C. these microorganisms are known as psychotropic pathogens (i.e. could tolerant disease causing). This is a serious concern because it means that even good refrigeration cannot always be assumed to protect foods. Below -9.5C there is no significant growth of spoilage or pathogenic microorganisms in food; instead there is a gradual decrease in the numbers to living organisms. But, as pointed out previously, destruction of microorganisms by freezing is not complete; when the food is thawed, there can be rapid multiplication and spoilage.
REFRIGERATION AND COOL STORAGE
Refrigeration and cool storage in general is the gentlest method of food preservation. It has relatively few adverse effects on the taste, texture, nutritive value, and other attributes of foods, provided simple rules are followed and storage periods are not excessive. One cannot say this of heat, dehydration, irradiation, and other methods of preservation, which often immediately result in changes in food, however small.
Although refrigeration and cool storage reduces the rate of food deterioration, with most foods it will not prevent deterioration to anywhere the same degree as does heat, dehydration, irradiation, fermentation, or true freezing. Table 9.1 indicates the generally useful storage life of plant and animal tissues at various temperatures. At 0C, which is lower than most commercial or household refrigerators, the life of such perishables as animal flesh, fish, poultry, and many fruits and vegetables is generally less than 2 weeks. At the more common refrigerator temperature of 5.5 C, storage life is often less than 1 week. On the other hand, these products held at 22 C or above may spoil in 1 day or less.
Ideally, refrigeration of perishables starts at time of harvest or slaughter and is maintained throughout transportation, warehousing, merchandising, and storage prior to ultimate use. This is not required from the standpoint of microbial spoilage alone but also to maintain the flavor, texture, and other quality attributes of many foods.
A few hours’ delay between harvest or slaughter and refrigeration is sufficient to permit marked food deterioration to occur. This is particularly true with certain metabolically active fruits vegetables. These not only will generated heat from respiration but will convert metabolites from one form to another. The loss of sweetness from sweet corn is an example of the latter. At 0 C sweet, corn can still metabolize its own sugar, but generally less than 10 % is lost in 1 day and 20% in 4 days. At 20 C, however, these losses can amount to 25% in 1 day and can far exceed this on a hot summer afternoon. To minimize such losses, cooking systems are brought into the harvest field. Figure 9.2 shows one type of portable cooler. As they are picked, fruits or vegetables pass through this hydro-cooler where they are sprayed with jets of cold water. The water also may contain a germicide to inactivate surface microorganisms. The cooled produce is then loaded into refrigerated trucks or railroad cars in route to refrigerated warehouses.
Quick cooking dies not simply mean immediate placement of bulk foods into a refrigerated railroad car or warehouse in all cases. Cooking is the taking of heat out of a body. If the body is large, the time to remove sufficient heat can be so long as to permit considerable food spoilage before effective preservation temperatures are reached. The hydro cooler of Fig. 9.2 accomplishes rapid cooking aided by subdivision of the produce as it is fed through the machine. Similarly, subdivision of bulk produce favors cold air circulation in refrigerated storage rooms. Leafy vegetables may be quickly cooled by spraying them with water and creating a vacuum to promote evaporative cooking. Current use of cold nitrogen gas from evaporating liquid nitrogen in refrigerated trucks, railcars, and ship holds also aids in providing intimate cold contact and quick cooling of produce. This has the further advantage of displacing air from the refrigerated area, which can be beneficial for certain products. Bulk liquids are best cooled rapidly by passing through an efficient heat exchanger before putting them into refrigerated storage. Animal carcasses at time of slaughter are at a temperature of about 38 C, which must be lowered to about 2 C in less than 24 h if quality is to be maintained.
Requirements of Refrigerated Storage
The principal requirements for effective refrigerated storage are controlled low temperature, air circulation, humidity control, and modification of gas atmospheres.
Controlled Low Temperature
Properly designed refrigerators, refrigerated storage rooms, and warehouses will provide sufficient refrigeration capacity and insulation to maintain the room within about ± 1C of the selected refrigeration temperature. In order to design a refrigerated space capable of maintaining this temperature, it is necessary to know, in addition to the insulation requirements, all factors that may generate heat within this space or the influence ease of removal of heat from the space. These factors include the no of heat-generating electric lights and electric motors that may be operating the number of people that may be working in the refrigerated space, how often doors to the area will be stored in the refrigerated area.
This latter item is of importance for two major reasons: first, the quality of heat that must be removed from any amount of food to lower it from one temperature to another is determined by the specific heat of the particular food; and second, during and after cooling, such foods as fruits and vegetables respire and produce their own hat at varying rates. Both the specific heats and respiration rates of all important foods are known or can be closely estimated. These values, in addition to the items mentioned above, are necessary to calculate the “refrigeration load,” which is the quality of heat that must be removed from the product and the storage area in order to go from an initial temperature to the selected final temperature and then maintain this temperature for a specified time.
The heat evolved during respiration by representative fruits and vegetables is listed in Table 9.2. The amount of heat produced varies with each product, and like a metabolic activity decreases with storage temperature. Products with particular high respiration rates, such as snap beans, sweet corn, green peas, spinach, and strawberries, are particularly difficult to store. Such products, if closely packed in a bin can rot in the center even when the surrounding air is cool due to the heat generated by the product. The relationships between specific heats of foods and calculations refrigeration load will be discussed in the section on freezing and frozen storage.
Air Circulation and Humidity.
Proper air circulation helps move heat away from the vicinity of food surfaces towards refrigerator cooling coils and plates. But the air that is circulated within a cold storage room must not be too moist or too dry. Air of high humidity can condense moisture on the surface of cold foods. If this is excessive, molds will grow on these surfaces common refrigeration temperatures. If the air is too dry, it will cause drying out of foods. All foods are different with respect to supporting mold growth and tendency to dry out, and so for each, an optimum balance must be reached. The optimum relative humidity (RH) to be maintained in cool storage rooms for most foods is known. Table 9.3 summarizes the best storage temperatures and relative humidities for many food items and their approximate storage life. (This table also includes data necessary calculation of refrigeration loads). Most foods store best at refrigeration temperature, when relative humidity of air is between about 80% and 95%. The optimum relative humidity for a particular food is generally related to its moisture content and the eases with which it dries out. For example, celery and several other crisp vegetables reduced a relative humidity 90-95%, whereas nuts may do well at only 70%. On the other hand, dry and granular products such as powdered milk and eggs, which have extended storage lives at refrigeration temperatures, are favored by very dry atmospheres, and relative humidities above about 50% can cause excessive lumping and caking if the packaging is not moisture tight.
When refrigerated storage is to be for prolonged periods, various techniques are used to maintain quality. Foods that tend to lose moisture can be protected by several packaging methods. This is important since otherwise there would be a continual migration of moisture from the food to the storage atmosphere and onto refrigerated coils and plates since moisture vapor tends to condense on cold surfaces.
Beef that is tenderized by ageing in cool rooms often presents a problem. Conventionally, ageing id done at about 2 C for a period of several weeks. If the relative humidity of the storage room is much below 90%, the beef dries out; if it is above 90%, the beef will mold. Precise control of relative humidity is difficult to achieve. To retard mold growth and the development of surface bacterial slime, ultraviolet light is sometimes employed. In one type of particular accelerated ageing process beef is aged in 2 or 3 days by combining high humidity with a temperature of about 18 C. this also speeds surface microbial growth, which is kept in check by ultraviolet light. In applications such as this, the dosage of ultraviolet irradiation must be controlled, as excessive exposure to ultraviolet light can cause surface fat to become rancid.
Modification of Gas Atmospheres
Controlled-atmosphere (CA) storage is used for apples and other fruits in order to inhibit over ripening in cold storage. Stored fruits and vegetables consume oxygen and give off carbon dioxide during storage. Three ways to slow down this respiration and the physiological changes that accompany it are reducing temperature, reducing but not eliminating oxygen, and increasing the carbon dioxide. The optimum temperatures, relative humidities, and gas compositions of the atmosphere differ for different fruits and even for varieties of the same fruits. In the case of McIntosh apples, optimal conditions include a temperature of 3 C, 87% relative humidity (RH), and an atmosphere containing 3% oxygen (normally, 21%), 3% carbon dioxide (normally 0.03%) for about 1 month then 5% carbon dioxide, and nitrogen to make up the balance. In practice, a cold storage warehouse is made gas-tight, brought to temperature, filled with fruit, and sealed. Commercial gas generators then replace air with the chosen gas atmosphere and may also introduce water vapor to maintain the desired relative humidity. The warehouse is usually sealed for months until it is to be emptied. If someone must enter the warehouse to make repairs, an oxygen mask is required. Under these conditions, apples retain quality in storage for better than 6 months.
Controlled-atmosphere storage is not limited to storage in a warehouse. In a sense, CA storage is practiced whenever food is sealed in a package under vacuum, nitrogen, carbon dioxide, or any other departure from the composition of air. In recent years, the concept of controlled atmosphere packaging has become widely used for individually packaged foods. Perishable foods such as meats, high-moisture pasta, fish, and fresh fruits and vegetables can be sealed in packages in which the air has been replaced with some mixture which extends shelf life. High-moisture-content pasta products are common examples of such technologies. These technologies require the use of strict control of quality and storage temperatures in order to ensure the safety and quality of such products.
Other examples of controlled-or modified-atmosphere storage include use of antimicrobial vapors or fumigants to control molds and use of ethylene gas to speed ripening and color development of citrus fruits and bananas. The fact liquid-nitrogen cooking displaces air with nitrogen gas has already been mentioned, and considerable research has been done to determine the full potential of this kind of modified-atmosphere storage.
Since animal as well as plant tissues consume and give off gases, it would be expected that gas equilibria would affect many food properties. This certainly is so with respect to the pigment changes of red meat, the growth and metabolic patterns of surface ripening as well as spoilage microorganisms, and the staling rate of cold storage eggs. In the latter case, besides oil coating to minimize water and carbon dioxide losses, eggs have been stored in warehouses enriched with carbon dioxide to minimize loss of this gas which is associated with egg PH and freshness.
The term hypobaric storage has been used to describe another type of CA storage. In this case the refrigerated storage area is maintained under reduced pressure and high humidity. This decreases the amount of air, and with it the amount of oxygen in the area, while the high humidity prevents product dehydration. Hypobaric storage has been used in warehouses as well as enclosed truck bodies. Since altered gas equilibria can change the rates of microbial and enzymatic spoilage, the possibilities for energy savings (less intense refrigeration) through use of modified atmosphere is of increasing interest.
Changes in Food During Refrigerated Storage
The deterioration of foods during cool storage are influenced by the growing conditions and varieties of plants, feeding practices of animals, conditions of harvest and slaughter, sanitation and damage to tissues, temperature of cool storage, mixture of foods in storage, and other variables.
For example, Florida grapefruits store well at 0C, whereas Texas March grapefruits store better at 11C. McIntosh apples store well at 2-5C, but Delicious apples do better at 0C. Pigs fed on substances high in unsaturated fats such as peanuts and soybeans produce softer pork and lard than the same animals fed on cereal gains; meat from the latter keeps better in cold storage. Animals-permitted to rest before slaughter build up glycogen (animal starch) reserves in their muscles. Following slaughter, this is converted to lactic acid, which is a mild preservative and enhances the keeping quality of meat in cold storage. Animals that are exercised or excited before slaughter use up their glycogen reserve, less is available for conversion to lactic acid, and keeping quality is impaired.
Too low refrigeration temperature can cause damage called “chill injury” to fruits and vegetables even when these are not physically damaged by freezing (see Table 7.4). This is not surprising since living plants would be expected to have optimum temperature requirements just as animals do. Many of the defects listed in Table 7.4 are of microbial origin, reflecting a weekend physiological state and a decrease in resistance to this kind of deterioration. In the case of bananas and tomatoes on the other hand, storage temperature below about 13C slow down the activities of natural ripening enzymes and result in poor colors. Nevertheless, for the majority of perishable foods, no cooking at all generally would be far worse than refrigeration temperatures that are somewhat too low.
Refrigerated storage permits exchanges of flavors between many foods. Butter and milk will absorb odors from fish and fruits, and eggs will absorb odors from onions. It is best to store different foods, especially odorous ones, separately, but this is not always economically feasible. In many instances, odor exchange can be prevented by effective packaging.
The previously mentioned losses of sugar in sweet corn stored at refrigeration temperatures are due to synthesis of starch from the sugar and, thus, do not represent actual nutrient losses. However, some changes that occur in foods during refrigerated storage represent true nutrient losses. An important example is loss of vitamin C and other vitamins, which is common in many foods held for relatively short periods under refrigeration (Table 9.4).
Still other common changes during refrigerated storage involve loss of firmness and crispness in fruits and vegetables, changes in the colors of red meats, oxidation of fats, softening of the tissues and drip page from fish, staling of bread and cake, lumping and caking of granular foods, losses of flavor, and a host microbial deteriorations often unique to a specific food and caused by the dominance of a particular spoilage organism. Some foods should not be refrigerated. Bread is an example. The rate of staling of bread is greater at refrigeration temperatures than it is at room temperature.
Staling can be arrested by freezing. These and other difference between foods at refrigerated temperatures cause the storage requirements indicated in Table 9.3
Benefits other than Preservation
In the food industry, cooking generally is used for its preservation value. There are many situations, however, where cooking provides other advantages and improves the processing properties of foods. Cooking is employed to control the rates of certain chemical and enzymatic reactions as well as the rates of growth and metabolism of desirable food microorganisms. This is the case in the cool ripening of cheeses, cool ageing of beef, and cool ageing of wines. Cooking also improves the ease of peeling and pitting peaches for canning. Cooling improves the ease and efficiency of meat cutting and bread slicing. Cooking precipitates waxes from edible oils. Water for soft drinks is cooled before carbonating to increase the solubility of carbon dioxide. More will be said about these applications of cooling in later chapters.
Economic Considerations
Where cooling is used for preservation in a multiproduct warehouse, supermarket, or household refrigerator, it is not always economical or practical to separate foods and provide each with the optimum temperature and humidity. As a compromise, the refrigerated area often is held somewhere within the range 2-7C with no special provisions made to control humidity. Even under these conditions, refrigeration significantly improves the safety, appearance, flavor, and nutritional value of our food supply. It further reduces losses from insects, parasites, and rodents.
FREEZING AND FROZEN STORAGE
As a preservation method, freezing takes over where refrigeration and cool storage leave off. Freezing has been a major factor in bringing convenience foods to the home, restaurant, and institutional feeding establishments. Because freezing, properly done, preserves foods without causing major changes in their size, shape, texture, color, and flavor, freezing permits much of the work in preparing a food item or an entire meal to be done prior to the freezing step. This transfers operations that formerly had to be done in the home or restaurant to the food processor. Such diverse items as chicken pot pie, breaded fish sticks, whole entrees, whipped topping, chiffon and fruit pies, and complete dinners are, today, commonly frozen. The wide array of available frozen food products, many sold in their final serving dishes, represents a major revolution in the food industry and reflects gross changes in eating habits. There are more meals being eaten outside the home than ever before. This includes meals in restaurants, colleges, school lunch programs, hotels, airplanes, hospitals, and so on. Labor costs are steadily rising, forcing maximum use of convenience foods in food-handling establishments.
At present, no form of food preservation is as well suited to provide maximum convenience as freezing. Although dehydrated foods offer convenience, they require reconstitution on an individual component basis to satisfy varying water needs, and then also require heating. Not so with frozen foods. Many items can be completely prepared and assembled together for a single thawing-heating operation. Quality, of course, rests on well-developed scientific principles.
Initial Freezing Point
It is a basic property of aqueous solutions that increasing their concentrations of dissolved solids will lower their freezing points. Thus, the more salt, sugar, minerals, or proteins in a solution, the lower its freezing point and the longer it will take to freeze when put into a freezing chamber. If water and fruit juice, for example, are placed in a freezer, the water will freeze first. Further, unless the temperature is considerably below the freezing point of pure water, the juice will never freeze completely but rather will become icy and slushy. What is happening is that the water component of the juice freezes first and leaves the dissolved solids in a more concentrated solution which requires a still lower temperature to freeze it.
Since different foods have quite different water contents and kinds and amounts of solids dissolved in the water, they have different initial freezing points (Table 9.3) and, under a given freezing condition, require different times to reach a solidly frozen state. This alone provides much of the explanation of why cultivars of the same fruit or vegetable, which have somewhat different compositions, behave differently on freezing. Even the same cultivar grown under different irrigation and fertilization practices will exhibit variations in composition, including differences in mineral content absorbed from the fertilizers. For this reason, frozen food producers who want to have strict control over the freezing processes specify the cultivar to be grown and may even supply seed and fertilizer to help guarantee controlled composition and other properties of raw materials.
Freezing Curve
A given unit of food will not freeze uniformly; that is, it will not suddenly change form liquid to solid in the case of milk placed in a freezer, the liquid nearest the container wall will freeze first, and the first ice crystals will be pure water. As water continues to be frozen out, the milk will become more concentrated in minerals, proteins, lactose, and fat. This concentrate, which gradually freezes, also becomes more concentrated as freezing proceeds. Finally, a central core of highly concentrated unfrozen liquid remains; if the temperature is sufficiently low, this central core also will ultimately freeze
The freezing point of pure water is 0 C, but, actually, water does not begin to freeze at 0C. instead, it generally becomes super cooled to a temperature several degrees below 0C before some stimulus such as crystal nucleation or agitation initiates the freezing process. When this occurs, there is an abrupt rise from the super cooled temperature to 0 C due to the evolution of the latent heat of crystallization. Even if the water is in an environment far below 0 C, as long as free water is freezing and giving up latent heat of crystallization or fusion the temperature of a pure water –ice mixture will not drop below 0 C. Only after all of the water has frozen will the system drop of the freezing environment.
Much of this is true also for food systems containing water, but since foods contain dissolved solids, progressive freezing is somewhat more complex. The freezing curve for a thin section of beef shown in Fig. 9.3 was obtained by placing the beef in a freezing chamber which was below -18 C and recording the temperature of the beef with time as it froze. At the same time, the percentage of water that was converted to ice was determined as a function of temperature or time. As the beef is chilled, it first drops from its initial temperature to a super cooled temperature below its freezing point. Nucleation or agitation initiates formation of the first ice crystal, and latent heat of fusion causes the temperature to rise to the freezing point, which is just below 0 C because of the dissolved solids in the water phase.
If this were the freezing curve of pure water, it would not drop below the freezing point as long as liquid water remained. In the case of beef and other foods, however, the temperature continues to drop as more and more water is frozen. This is largely because as more water is frozen out, the concentration of solutes in the remaining water progressively increases and exerts a greater and greater freezing point depression on the remaining solution.
It also should be noted from Fig 9.3 that at about -4C some 70% of the water is frozen, and the beef would appear solidly frozen; however, at -9.4C, about 3% of the water still remains unfrozen, and even at -18C, not all of the water is completely frozen. These small quantities of unfrozen water are highly significant, particularly since within them are dissolved food solutes which are concentrated and are therefore more prone to reaction with one another and with other food constituents.
Since the compositions of foods differ, they have characteristic freezing curves differing somewhat in shape. Generally, one can identify the zone of super cooling, the inflection up to the freezing point, and the subsequent drop in temperature if there is a sufficient temperature differential between the freezing food and the freezer environment. This differential provides the driving force for continued heat transfer out of the food.
Changes During Freezing
Water when frozen can burst iron pipes, so it should not be surprising that unless properly controlled, freezing can disrupt food texture, break emulsions, denature proteins, and cause other changes of both physical and chemical nature. Many of these changes are related to food composition, which, in turn, is influenced by agricultural practices long before the freezing process.
Concentration Effects
For quality to be maintained in frozen storage, most foods must be solidly or very nearly solidly frozen. An unfrozen core or a partially frozen zone will deteriorate in texture, color, flavor, and other properties. In addition to the possible growth of psychotropic microorganisms and to the greater activity of enzymes when water remains unfrozen for deterioration in partially frozen food is due to the high concentration of solutes in the remaining water. Thus, when milk is slowly frozen, as can occur outdoors in winter, the concentration of minerals and salts can denature proteins and break fat emulsions, to cause curdling and butter granules. Flavor changes also occur.
Damage from the concentration effect can be of various kinds:
If solutes precipitate out of solution, as do excessive levels of lactose in freezing ice cream, a gritty, sandy texture to the food can occur.
Solutes that do not precipitate but remain in concentrated solution can cause proteins to denature because of a “salting out” effect.
Acidic solutes on concentration can cause the ph to drop below the isoelectric point (point of minimum solubility), causing proteins to coagulate.
Colloidal suspensions are in delicate balance with respect to the concentration of anions and cations. Some of these ions are essential to maintain colloids, and concentration or precipitation of these ions can disturb this balance.
Gases in solution also are concentrated when water freezes. This can cause supersaturating of the gases and ultimately force them out of solution. Frozen beer or soda pop may have such a defect.
The concentration effect can also cause a dehydration of adjacent tissues at the micro environmental level. Thus, when ice crystals form in extracellular liquid and solutes are concentrated in the vicinity of the ice crystals, water will diffuse from within the cells through the membranes into the region of high solute concentration to restore osmotic equilibrium. This shift of moisture is rarely completely reversed on thawing and can result in loss of tissue turgor.
Ice Crystal Damage
Solid foods from living tissues such as meats, fish, fruits, and vegetables are of cellular structure with delicate cell walls and cell membranes. Within and between the cells is water. When water freezes rapidly, it forms minute ice crystals, when it freezes slowly, it forms large ice crystals and clusters of crystals. Large ice crystals forming within or between cells cause much more physical rupture and separation of cells than do small crystals. The changes in the texture of strawberries resulting from freezing is an example. Large ice crystal damage is detrimental not only to cellular foods, but it also can disrupt emulsions such as butter, frozen foams like ice cream, and gels such as puddings and pie fillings. In the case of butte, ice crystals that grow within individual water droplets dispersed in the continuous fat phase can penetrate through the fat and merge. When such butter is later thawed, water pockets and water drip page result. In the case of ice cream, large ice crystals can puncture frozen foam bubbles. Such a condition leads to loss of volume on storage and during partial melting. Gels behave somewhat like butter, often exhibiting syneresis or water separation.
Rate of Freezing
Whether the concentration effect r physical damage from large ice crystals is the more detrimental during freezing and frozen storage depends on the particular food system under consideration. However, in either case, fast freezing is necessary for high quality.
Fast freezing produces minute ice crystals. It also minimizes concentration effects by decreasing the time concentrated solutes are in contact with food tissues, colloids, and individual constituents during the transition from the unfrozen to the fully frozen state. For these reasons, modern methods of freezing and freezing devices are designed for very paid freezing where high food quality can justify the cost. Generally, the faster the freezing rate, the better the product quality. However, from a practical standpoint, progressive freezing equivalent to about 1.3 cm (0.5in.) per hour are satisfactory for most products. This would mean that a flat package of food 5 cm in thickness and frozen rom both major surfaces should be frozen (to -18C or below) at its center in about 2h. Plate freezers easily do this, and liquid-nitrogen freezers may cut down the time to a few minutes. Unfortunately, home freezers can have one of the slowest rates of freezing.
Choice of Final Temperature
A consideration of all the factors –textural changes, enzymatic and no enzymatic chemical reactions, microbiological changes, and costs –leads to the general conclusion that foods should be frozen to an internal temperature of -18C(0F) or lower and kept at -18C or lower throughout transport and storage. Economic considerations generally preclude temperatures below about –30C during transport and storage, although many foods commonly are frozen to temperatures below this in an effort to achieve the advantages of rapid freezing.
The choice of –18C or below as the recommended temperature for freezing and storage is based on substantial data and represents a compromise between quality and cost. Microbiologically, -18C storage would not be strictly required since pathogens do not grow below about 3.3C and normal food spoilage organisms do not grow below -9.5C. On the other hand, transportation and frozen storage facilities are expected to vary somewhat at any chosen temperature setting. Therefore, the use of -18C provides a reasonable measure of safety against food spoilage organisms and a still greater margin of safety against pathogens; and, indeed, frozen foods have enjoyed an excellent public health record over the years.
For control of enzymatic reactions, -18C is not exceptionally low since some enzymes retain activity even at -73C, although reaction rates are extremely slow. Enzyme reaction rates are faster in super cooled water than in frozen water at the same temperature. In most foods there remains considerable unfrozen water at -9.5C, and long term storage at this temperature results in severe enzymatic deterioration of food quality, especially of an oxidative nature. Storage at -18C sufficiently retards the activity of many food enzymes to prevent significant deterioration. In the case of fruits and vegetables, the enzymes are inactivated prior to freezing by heat blanching or chemical treatment.
Non enzymatic chemical reactions are not entirely stopped at -18C, but proceed very slowly. In the freezing zone, the generalization that reaction rates are approximately halved for every 10C drop in temperature does not hold well, since many reactions proceed in solution, and in this zone, solutions concentration is changing rapidly with the freezing of water. Nevertheless, the lower the temperature, the slower the reactions rates and the less unfrozen water present to serve as solvent of chemical reactants.
The overall effects of low temperature on long-term storage of various foods are indicated in Table 9.5. Many vegetables, fruits, and non-fatty meats, if properly packaged and frozen, retain good quality during storage at -18C for 12 months or longer most fish are less stable. At higher temperature of -9 to -7C, quality may be retained for periods of only days or a few weeks, depending on product.
Quality and subsequent storage life would be still better for many foods if they were frozen and stored well below -18C. It is relatively easy to freeze foods to -30C and even lower by several methods, and the costs are not excessive. What is more difficult and very expensive, however, is maintaining food at or below -30C during transport and warehouse or supermarket cabinet storage. Many refrigerated trucks on the road today are not capable of holding a temperature of even -18C, and supermarket display cases often are above -18C near the top, although they may be colder below.
Damage from Intermittent Thawing
The kinds of damage that can occur to foods during slow freezing also occur during slow thawing. Repeated freezing and thawing cycles are very detrimental to stored foods. Repeated thawing need not be complete for damage. Complete thawing in storage is rare and generally occurs only when there is complete breakdown of the cold storage equipment. This is easily recognized and quickly corrected. However, all commercial frozen food distribution or storage systems have a measurable temperature cycle. Such cycles are part of the temperature control systems, and it is not uncommon for a frozen storage chamber to go from its maximum to its minimum temperature and back again on roughly a 2-h cycle. This could mean 360 cycles a month and over 4000 cycles a year.
As little as a 3C fluctuation in freezer storage temperature above and below the -18C mark can be damaging to many foods. Above -12C, thawing intensifies the bathe unmelted crystals, causing them to grow in size. Whatever the temperature fluctuation in the storage area, because heat transfer has a finite rate there will be a lag effect in the food itself and the food generally will experience less of a temperature range than the room or cabinet. Nevertheless, room or cabinet temperature variations of greater than a few degrees from the -18C mark over a period for weeks or months will noticeably damage the quality of most frozen foods, and such freezer storage facilities should be repaired.
Frozen foods being thawed for ultimate use also are subject to quality loos, especially if thawing is slow. Once again, concentration effects can occur. Brought to the same temperature, the most concentrated solutions that freeze last are the first to thaw. These commonly are eutectic mixtures. A eutectic mixture is a solution of such composition that it freezes (or thaws) as such rather than becoming more concentrated due to further separation of pure ice. In other words, the eutectic mixture freezes (and thaws) as a mixture, and the frozen eutectic will have a constant proportion of ice crystals intermingled with solute crystals. The temperature at which a eutectic mixture is formed is called the eutectic temperature or eutectic point. A dilute solution of NaCl in water under freezing conditions will first freeze out pure water and become more concentrated in NaCl. At -21C. the remaining water and salt would consist of a mixture of 23% NaCl and 77% water. This would freeze into eutectic ice of the same composition. Where ice from pure water has a melting point of 0C, eutectic ice could be removed from the system and would have a melting point of -21C, and, therefore, such ice would be a better refrigerant than pure water ice and has been used as such commercially.
Food materials are complex mixtures and pass through several eutectic compositions in the course of becoming solidly frozen and on thawing. If thawing is slow, there is more time for food constituents to be in contact with concentrated eutectic mixtures and damaging concentration effects are intensified.
Another reason why quick final thawing is superior to slow thawing is illustrated in Table 9.6. Large volumes of frozen food, such as a 30-lb can have frozen whole egg, can take 20-60 h to thaw in air, depending on the air temperature. Cool running water and other techniques can markedly reduce this time. Since bacteria survive the freezing process, when thawing times are long and temperatures of the product rise, there is an opportunity for bacterial multiplication.
Refrigeration Requirements
A product’s refrigeration load is the quantity of heat that must be removed to reduce the temperature of the product from its initial temperature to the temperature consistent with good frozen food storage. If the food is cooled from above its freezing point to a storage temperature below its freezing point, then quantitatively this refrigeration load is made up of three parts: the number of heat units that must be removed.
Cool the food from its initial temperature to its freezing point.
Cause a change of state at the freezing point.
Lower the temperature of the frozen product to the specified storage temperature.
These heat units may be calculated in terms of British Thermal Units (Btu), calories (cal), or joules (J). Much of the original work in refrigeration and freezing was done using the British system of units, which has dominated the literature. This will gradually be replaced by the International System of Unites (SI), which employs joules and grams as the units of heat and weight.
Definition and Heat Constants
A Btu is the quantity of heat that will raise or lower the temperature of 1 lb. of water 1 F through the range 32-212F at normal atmospheric pressure. A calorie is the amount of heat that will raise or lower the temperature of 1 g of water 1 C (from 14.5 to 15.5C) at normal atmospheric pressure. (1 Btu = 252 calorie = 1055 joules or 1.055 Kj.)
Different substances can absorb different amounts of heat and are said to have different heat capacities. The heat capacity of water in Btu/ (lb. F) or 1 cal/(gc).
The specific heat of any substance is the ration of its heat capacity to that of water. Specific heat is a ratio like specific gravity and is independent of whether measurements are made in Btu or calories. In either case the specific heat of water is taken as the standard and is given a value of 1. Thus, in the British system the specific heat of a substance is the ratio of the heat required to raise or lower the temperature of a unit mass of the substance 1 F compared to the heat required to raise or lower the temperature of a unit mass of water 1 F. since the specific heat of water is 1, it follows that the specific heat of any substance is the amount of heat in. Btu required to raise or lower the temperature 1 lb. of the substance 1 F. in like fashion, this specific heat of any substance is the amount of heat in calories required to raise or lower the temperature of 1 g of the substance 1 C.
There are two types of heat: sensible heat and latent heat. Sensible heat is readily perceived by the sense of touch and produces a temperature rise of fall as heat is added or removed from a substance. Latent heat is the quantity of heat required to change the state or condition under which a substance exists, without changing its temperature. Thus, a definite quantity of heat must be added to ice at 0C, the same amount of heat must be added to ice at 0C to change it to water 0C. this is known as the latent heat of fusion or crystallization. Similarly, is going from water at 100C to steam at 100C, the latent heat of evaporation must be added to the system. The latent heat of fusion, which must be removed during freezing, is 144 Btu/lb. for water. The specific heat of a material is different in the liquid state and in the frozen stage; that is, a different number of Btu/lb. is required to raise or lower the temperature of a material 11 F depending on whether the material is above or below its freezing point.
The specific and latent heats of foods are used to determine refrigeration requirements for cooling, freezing, and storage. Typical values for a few foods are given in Table 9.7. Additional values can be found in several handbooks and in Table 9.3.
Calculation of Refrigeration Load
As indicated in Table 9.7, water has a specific heat before freezing of 1.00, a latent heat of fusion of 144, and a specific heat after freezing of 0.48. Therefore, if we wanted to freeze a pound of water from 60 F to 0F, we would have to remove 1 Btu/F from 60F down to 32F, plus 144 Btu to go from water to ice at 32F, plus 0.48 Btu/F from 32F down to 0F. This would be equal to 28+144+15 or 187 Btu.
Using the same method, the following general equations can be used to calculate the heat to be removed to cool and freeze any quantity of food material from any starting temperature to any frozen storage temperature, provided the freezing point of the material is known:
H1=SLW (T1 - TF), H1 = SSW(TF-TS)
H2 = HFW HFS = H1+H2+H3
Thus, H1 is the number of Btu required to cool the food from its initial temperature to its freezing point and is equal to SL (the specific heat of the food above its freezing point) X W (its weight in pounds) X Ti-Tf (the difference between the initial temperature and the freezing point in F).
H2 is the number of Btu required to change the food from the “liquid” state to the frozen state at its freezing point and is equal to HF (the latent heat of fusion of the food) X W (its weight).
Food Composition
Like metals and other materials, food constituents have different thermal conductivity properties which change with temperature. The greater the conductivity, the greater the cooking and freezing rates –all other things being equal. In the cooling and freezing temperature range, heat conductivities of water change little until the phase change from water to ice occurs. Since the thermal conductivity of ice is far greater than of water, the thermal conductivity of a food increases rapidly as it passes from the unfrozen to the frozen state. It should also be noted that fat has a much lower thermal conductivity than water, and air has a thermal conductivity far less than that of water or fat.
Several generalizations can be made about the effect of the composition of a food product on freezing rate under controlled freezing conditions. First, high levels of fat or entrapped air tend to reduce the rate of freezing. Second, rates of cooling and freezing are not constant during these processes since thermal conductivities change as water changes to ice. In addition, the physical structure of foods influences freezing rates. For example, if two food systems both contained 50% fat and 50% moisture, but one was an oil-in-water emulsion and the other was a water-in-oil emulsion, the two foods would be expected to have different thermal conductivity properties. The oil-in-water emulsion, with water being the continuous phase, should have greater thermal conductivity values at different temperatures than the corresponding water-in-oil emulsion of the same chemical composition. Other things being equal, the oil-in-water emulsion should freeze at a faster rate than the water-in-oil emulsion. Similarly, cuts of meat should conduct heat at different rates depending on whether the meat ins in contact with a refrigerated surface in a direction parallel or perpendicular to the layers of fat and onto the direction of orientation of the muscle fingers. We can make educated guesses as to how heat transfer will be affected by these variations, but unfortunately there is little published work on these food systems.
Non compositional Influences
The effects of various other factors (e.g., air velocity, product thickness, agitation, degree of contact between food and cooling medium, and packaging) on freezing rate are well known, follow the simpler rules of heat transfer, and largely determine the design of freezer systems. It is easy to cite the directional effects of such variables on freezing rate, and these will apply in virtually any system design. However, a quantitative measure of the effects of these variables generally must be established experimentally for each different food and system geometry to have validity.
The following principles apply in any system:
The greater the temperature difference between the food and the refrigerant, the faster the freezing rate;
The thinner the food piece or greater the heat transfer rate of the food package, the faster the freezing rate;
The greater the velocity of refrigerated air or circulating refrigerant, the faster the freezing rate;
The more intimate the contact between the food and the cooling medium, the faster the freezing rate;
And
The greater the refrigerating effect or heat capacity of the refrigerant, the faster the freezing rate.
In this latter case, if the refrigerant is a liquid that expands to a gas, then the refrigerant does not undergo phase change, such as salt brine, then its refrigerating effect is determined by its heat capacity or specific heat. More will be said about the refrigerating effect under liquid-nitrogen freezing.
The magnitude of the influence of these major variables on freezing rate can be substantia. Thus, lowering air temperature in a tunnel-type freezer from -18 to -30C can shorten freezing time of small cakes from 40 min to about 20 min. spraying with liquid nitrogen at -196C would cut freezing time to under 2 min. lowering of freezer temperatures to very low levels, however, does not give a straight-line freezing rate response; thus, the increase in freezing rate as temperatures decrease tends to drop off, especially at freezer temperature below about -45C.
In still air at -18C, small items such as individual fruits or small fish fillets may freeze in about 3h. increasing the air velocity at this temperature to 1.25 m/sec (250ft/min) will decrease freezing time of these items to about 1h. whereas an air velocity of 5 m/sec will further decrease freezing time to about 40 min. whether in cold air or in any other flowing refrigerant, increased velocity speeds freezing by carrying heat away from the surface of food and rapidly replacing warmed refrigerant with cold refrigerant to maintain the maximum temperature difference between the food and the refrigerant. However, freezing rate does not increase linearly with air velocity.
The effect of food or package thickness on freezing rate is such that when common packages are doubled from about 5 to 10 cm (2-4 in.) in thickness, freezing time increases about 2.5-fold. The slope of the thickness curve is such that as product thickness is increased further, the rate of increase in freezing time goes up faster than the rate of change in thickness. Thus, 13.6-kg (30-lb) cans of eggs or fruit may require 48-72 h to become thoroughly frozen. Commercial 0.21-m3 (55-gal) drums of fruits juices may require more than a week to freeze unless the juice is first slush-frozen by being passed through a heat exchanger prior to being filled into the large drums.
Freezing Methods
There are three basic freezing methods in commercial use: freezing in air, freezing by indirect contact with the refrigerant, and freezing by direct immersion in a refrigerating medium. Each method can be subdivided in various ways, as indicated in Table 9.8.
Cold air may be used with various degrees of velocity, progressing from still-air “sharp” freezing to the high-velocity blast freezer tunnel. The velocity of air also may be used to subdivide and move particles of materials to be frozen, as in the case of fluidized-bed freezing.
Indirect contact freezing includes those methods in which the food or the food package is in contact with a surface hat, in turn, is cooled by a refrigerant, but the food or food package does not contact the refrigerant directly. In the case of solid foods or foods in containers, this most commonly involves providing a flat surface in contact with refrigerated plates, which may contact one or two surfaces of the food or package.
In the case of liquid food and purees, the food is pumped through a cold wall heat exchanger and frozen to the slush condition.
Immersion freezing involves direct contact of the food or package with the refrigerant either by submerging the food or spraying the cold liquid onto the food or package surface.
With the exception of still-air “sharp” freezing, all of these methods are referred to as fast freezing methods and may be engineered for batch, semicontinuous, or continuous operation.
Air freezing
The oldest and least freezing method from an equipment standpoint is still-air “sharp” freezing. Here the food is simply placed in an insulated cold room at a temperature usually maintained in the range of -23 to -30C. The method was introduced around 1860 and became termed “sharp” freezing, since anything below -18C was then considered a very low temperature. Although there is some air movement by natural convection, and in some cases gentle air movement is promoted by placing circulating fans in the room, the method is essentially a still-air freezing method to be clearly distinguished from air-blast freezing, which employs air velocities that may exceed hurricane speeds. Depending on size of food items or packages and degree of separation between units, freezing time can be several hours to several days. Sharp freezing remains, today a very important freezing method. Sharp freezing conditions also are essentially the freezing conditions that exist in home freezers, except temperature commonly are closer to -18C than to -23 or -30C. Commercially, sharp freezers often double as frozen storage rooms where space permits.
Air-blast freezers typically are operated at temperatures of -30 to -45C with forced air velocities of 10-15 m/sec (20000-30000 ft./min). Under such conditions, 30-lb cans of eggs or fruit, which take 72 h to freeze thoroughly in a sharp freezer, may be frozen in 12-18 h. Blast freezers are of many designs, from rooms where food is frozen as a batch to tunnels through which carts or a belt may be moved continuously. The tunnel blast freezer shown in Fig. 9.4 combines an overhead conveyor belt with a lower level track for moving food carts. Particulate unpackaged foods, such as loose vegetables, are automatically fed onto the moving belt whose speed is adjustable according to the required freezing time. Frozen product is dropped from the belt into a collection hopper at the opposite end of the tunnel. Independently, loose foods or packaged cartons loaded on the carts can be moved through the tunneled at a selected rate.
Other designs make use of vertical movement of food on trays. Trays of particulate products such as peas or beans are automatically moved upward through a cold air blast. Freezing time of such particulates in thin layers may be on the order of 15 min. Today, along with foods frozen as a single block in a package, vegetables and other particulate items. Such as shrimp, are individually quick frozen (IQF) so that they can be poured from a bag for greater convenience. Since there is some tendency for such particulates to stick together during freezing, product mechanically dislodged from trays may be passed through a breaker devise to disaggregate large clusters before being conveyed under cold air to packaging.
In air-blast systems, manufacturers have developed numerous patterns of cold air flow to pass over, under, or through the product. Frequently, the principle of countercurrent air flow is employed to bring the coldest air into contact with the already frozen product as it is about to leave the tunnel or column. In this way, freezing is progressive and there is no tendency for product to rise in temperature and partially thaw through the freezing process, as would be possible in a concurrent system, where coldest air enters with unfrozen product and tends to rise in temperature through the tunnel as product gives up heat and freezes.
Modern blast freezers also provide means to overcome the major drawbacks inherent in high-velocity air freezers. Whenever unwrapped food is placed in a cold zone, there is a tendency for the food to lose moisture, whether during freezing or after the food is frozen. In a freezer this can have two consequences: frosting over of refrigerated coils or plates, necessitating frequent defrosting to maintain heat exchanger efficiency; and drying out of food at its surface, resulting in the defect known as “freezer burn.” This situation is markedly accelerated in blast freezers where the air is moving at high velocity. When food is frozen and water vapor molecules are removed from the frozen surface by the dryer cold air, sublimation, a form of freeze-drying occurs; this is essentially what freezer burn is.
To minimize freezer burn, which produces unsightly food surfaces, nutrient loss, and other defects, two techniques are employed. One is the practice of prechilling food with air of high relative humidity at about -4C. Here the food partially frozen in the humid air under goes minimum moisture loss. Then the prechilled surface-frozen food is moved into a second colder zone where it is quickly finish-frozen. The rapid finish freezing in colder air provides minimum time for the already cold product to lose more moisture, which also decreases the defrosting requirement on freezer coils. The other technique is to wet the unpackaged food pieces in the prechilling zone so as to freeze a thin ice glaze around each food piece. The glazed will sublime slightly but will protect the underlying food from freezer burn. These techniques are employed in continuous last freezers by providing a series of humidity and temperature than the circulating air. The problems of freezer burn and frosted coils, of course, are markedly less when packaged foods are frozen.
In various air-type freezers, cold air is blown up through a wire mesh belt, that supports and conveys the product. This imparts a slight vibratory motion to food particles, which accelerates freezing rate. When the air velocity is increased to the point where it just exceeds the velocity of free-fall of the particles, fluidization occurs; this is called fluidized-bed freezing. The dancing-boiling motion of peas in a fluidized bed freezer is shown in Fig. 9.5. This motion not only subdivides the product and provides intimate contact of each particle with the cold air, but keeps clusters from freezing together and so is particularly well suited to production of frozen items in the IQF form. Freezing times commonly are in the order of minutes.
Several types of fluidized-bed freezers are in commercial use. The working of one continuous type are shown in Fig. 9.6. Particulate foods are fed by a shaker onto a porous trough at the right. The food may be prechilled and even moistened if it is desired to produce a frozen glaze around each particle. The high-velocity refrigerated air fluidizes the product, freezes it, and moves it in continuous flow from right to left for collection and packaging. An interesting feature of this unit, which can be seen in the diagram at the left in Fig. 9.6, is continuous and automatic defrosting. Air is blown via the fan through the cooling coils and up through the porous food trough. The cold air, now moistened from the food, is recirculated to conserve refrigeration. It tends to condense its moisture onto the cooling coils. But a spray of propylene glycol antifreeze is maintained over the cooling coils to melt ice as it would be formed. In this way the cooling coils are maintained at maximum operating efficiency. The glycol solution is bled off to an evaporator where the accumulated water is easily removed.
Indirect Contact Freezing
Although it is of course possible to place solid food directly onto the surface of a block of ice or a block of dry ice, this is rarely done commercially. Rather, the food is placed on plates, trays, belts, or other cold walls which are chilled by circulating refrigerant but separate the food from the refrigerant. Thus, the food or its package is in direct contact with the cold wall but in indirect contact with the refrigerant. This permits the use of refrigerants that might otherwise adversely affect the food or its package.
The more important indirect contact freezers are typified by the Birdseye Multiplate Freezer. This consists of a number of metal shelves or plates through which refrigerant is circulated. The food, usually as flat packages, is placed between shelves and there is provision after loading for applying pressure to squeeze the shelves into more intimate contact with the top and bottom of the packages for faster freezing. All is enclosed within an insulated cabinet. Depending on refrigerant temperature, package size, degree of contact, and the type of food, freezing time is 1 -2 h for commercial packages 4-5 cm (1.5-2.0 in.) thick. This unit is a batch freezer.
Quite similar are various automatic plate freezers. These have provisions for automatically loading shelves from the packaging line. As a shelf is loaded, it is moved into pressure contact with the preceding shelf and into an insulated zone where freezing proceeds. At the rear of the freezing zone, frozen packages are discharged one shelf at a time, and the empty shelves return to the loading position. The plate freezer shown in Fig. 9.7 operates in this fashion.
In all indirect contact freezing machines, efficiency is dependent on the extent of contact between the plates and the food. For this reason, packages should be well filled or slightly overfilled to make good pressure contact with the plates; solid compact products such as meat or fish fillets freeze more rapidly than shrimp or vegetables where the individual pieces are separated from one another by small air spaces.
Indirect contact freezers for liquid foods and purees are quite different. They generally take the form of the tubular scraped-surface heat exchangers previously descried (see Fig. 8.12), with refrigerant rather than steam on the side of the wall opposite the food. As is the case when this equipment is used for heating, the liquid food is pumped through the inside tube with its rotating shaft or mutator. This occupies most of the inner tube space, so the food is forced to pass through the remaining as a thin layer in contact with the cold wall. Scraper blades attached to and rotating with the mutator continually scrape the cold wall as food tends to freeze onto it. This speeds the freezing rate three ways:
1. It keeps the cold wall free of a frozen insulting food layer, which would minimize the temperature difference between the unfrozen food and the effective cold wall.
2. It shaves ice crystals from the cold wall as freezing progresses and these ice crystals seed the unfrozen portion of the food, promoting further freezing within the mass
And
The scraper blades (and mutator) keep the mass in motion, continually bringing new portions of food into contact with the cold wall. Freezing is virtually instantaneous, occurring in a matter of seconds. In this type of unit, freezing is never carried to completion, or else the frozen product would freeze in the tube and choke off continuous flow. Instead, the product is frozen to the slush condition, packaged, and then hard-frozen in an air-blast or immersions type freezer.
Immersion Freezing
In a strict sense, air freezing is a kind of immersion freezing. However, the term immersion freezing generally is applied to refrigerants other than cold air. The advantages of direct immersion freezing include the following:
There is intimate contact between the food or package and the refrigerant; therefore, resistance to heat transfer is minimized. This is particularly important with irregularly shaped food pieces to be frozen very rapidly, such as loose shrimp, mushrooms, and the like.
Although loose food pieces can be frozen individually by immersion freezing and air freezing, immersion freezing minimizes their contact with air during freezing, which can be desirable for foods sensitive to oxidation.
For some foods, the speed of immersion freezing with cryogenic liquids produces quality unattainable by any other currently known freezing method.
Direct immersion freezing places limitations on the refrigerants that may be used, especially if they are to come into contact with unpackaged foods. These refrigerants must be nontoxic, pure, clean, free from foreign tastes, odors, colors or bleaching agents, and so on. Similarly, when the food is packaged, nontoxicity and no corrosiveness to the packaging material are important. The refrigerants for immersion freezing are of two broad classes: low-freezing-point-liquids, which are chilled by indirect contact with another refrigerant; and cryogenic liquids, such as compressed liquefied nitrogen, which owe their cooling effect to their own evaporation.
The low-freezing-point liquids that have been used for contact with no packaged foods include solutions of sugars, sodium chloride, and glycerol. These must be used at sufficient concentration to remain liquid at -18C or lower to be effective. In the case of sodium chloride brine, for example, this requires a concentration of about 21%. Temperatures as low as -21C can be achieved with a 23% brine, but this is the eutectic point, and at lower temperatures a salt and water mixture freezes out of solution, so the lowest practical brine freezing temperature is -21C. brine cannot be used with unpackaged foods that should not become salty; today, brine for direct immersion freezing is largely restricted to freezing of fish at sea. Sugar solutions have been used to freeze fruits, but the difficulty here is that to remain liquid at a temperature of -18C, a solution of approximately two-thirds sucrose is required, which becomes very viscous at the low temperature. Glycerol and water mixtures have been used to freeze fruit, but, like sugar, cannot be used for foods that should not become sweet. One can get down to -47C with a 67% glycerol solution in water. Another low-freezing point liquid related to glycerol is propylene glycol. A 60% propylene glycol -40% water mixture freezes at -51C. propylene glycol is nontoxic but has an acrid taste. For this reason, its use in immersion freezing is generally limited to packaged foods.
Commercial equipment for immersion freezing with low-freezing-point liquids is typified by the continuous round shell direct immersion freezer which is well suited to the freezing of food in cans(Fig.9.8). The cans are positioned at the periphery of this rotating reel, and refrigerant is circulated in the annulus between the outer shell and the inner reel. Since the inner reel is closed at its ends, refrigerant flows only through the space occupied by the cans, reducing the volume of refrigerant needed. In a typical operation, about 400(6 oz.) cans are frozen per minute. Residence time or freezing time can be 30 min. rotation of the cans imparts motion to fluid foods in the cans as they freeze, contributing to a faster, more uniform, small ice crystal type of freezing.
Immersion Freezing with Cryogenic Liquids. Cryogenic liquids are liquefied gases of extremely low boiling point, such as liquid nitrogen and liquid carbon dioxide, with boiling points of -196C and -79C, respectively. Today, liquid nitrogen is the most commonly used cryogenic liquid in immersion freezing of foods.
The major advantages of liquid nitrogen freezing are the following:
1. It undergoes slow boiling at -196C, which provides a great driving force for heat transfer.
2. Liquid nitrogen, like other immersion fluids, intimately contacts all portions of irregularly shaped foods, thus minimizing resistance to heat transfer.
3. Since the cold temperature results from evaporation of liquid nitrogen, there is no need for aa primary refrigerant to cool this medium.
4. Liquid nitrogen is nontoxic and inert to food constituents. Moreover, by displacing air from the food it can minimize oxidative changes during freezing and through packaged storage.
5. The speed of liquid-nitrogen freezing produce frozen foods with a quality unattainable by no cryogenic freezing methods. Although many products do not require such fast freezing for good quality, some products do not require such fast freezing for good quality, some products such as mushrooms cannot be frozen by other methods without excessive tissue damage.
The major disadvantage of liquid-nitrogen freezing generally cited is its high cost. Some additional properties of liquid nitrogen deal with its heat capacity or refrigerating effect. In being vaporized from a liquid at -196C to a gas at -196C each kilogram of liquid nitrogen absorbs 200 Kj, the latent heat of vaporization. Then each kilogram of gas at -196C absorbs another 186 Kj in rising in temperature to -18C; this is the specific heat of the gaseous nitrogen times the temperature rises from -196 to -18C. Thus, the total heat uptake of the liquid at -196C going to -18C is 386Kj/kg.
This is very important in the design of spraying types of liquid-nitrogen freezers. To get the maximum freezing effect from spraying, the nitrogen should impinge on the food surface as liquid droplets rather than as a cold gas in order to achieve the cooling effect of the latent heat of vaporization plus the sensible heat of gas temperature rise, or 386J/kg of liquid. If the spray permits the liquid to vaporize before contacting the food, then a cooling effect of only 186J/kg of gas is achieved as the gas goes from a temperature of -196 to -18C.
The principles of manufacture and handling of liquid nitrogen should be understood. Liquid nitrogen is manufactured by compressing air and simultaneously removing the heat of compression. The cooled compressed air is then allowed to expand through specially designed valves. This expansion causes further chilling of the air to the point of liquefaction, producing a mixture principally of liquid nitrogen and liquid oxygen. Since liquid oxygen has a higher boiling point than liquid nitrogen, namely, -183C, the oxygen and nitrogen can be separated by distillation. Liquid nitrogen with its lower boiling point of -196C comes off first as a gas, which can be recompressed into a liquid. In recent years, technological advances in the production of liquid gases have stemmed from the needs of the space program where liquid oxygen is an important fuel. At -196C and at atmospheric pressure, liquid nitrogen boils gently. It does not produce an excessive pressure of nitrogen gas above the liquid if placed in a vessel and maintained at -196C; this is the key to liquid-nitrogen storage and handling. Liquid nitrogen is transported and stored at -196C by housing it in large insulated tanks of the thermos bottle or Dewar flask type. As long as insulation maintains this low temperature, pressure developed in the tank is comparatively small and not dangerous. In contrast, compressed nitrogen gas at room temperature is under great pressure and requires storage in the familiar high-pressure steel gas cylinders.
Manufacturers of liquid nitrogen deliver it in tank truck quantities to insulated storage tanks at food plants. Filling is done simply by hose connection from the tank truck, often making use of the slight pressure of nitrogen gas above the liquid in the tank truck to force the liquid nitrogen through the hose into the storage vessel. The same procedure is used to deliver liquid nitrogen from the storage vessel to the liquid-nitrogen food freezer or to a refrigerated truck for transporting frozen food (Fig. 9.9). The food freezing equipment commonly is of tunnel construction with a continuous mesh belt (Fig. 9.10). The earlier practice of submerging the food under liquid nitrogen has largely given way to more efficient use of liquid-nitrogen sprays. Design features are aimed at depositing the spray as a liquid onto the food surface and minimizing sensible heat lose through insulation; in very large installations, provisions may be engineered to recover and recompress the vaporized nitrogen for reuse. When liquid nitrogen contacts the relatively warm food, it boils violently. In most installations it is not recompressed, but the spent nitrogen gas, which still may be in the range of about -18 to 4C, is vented to contact and prechilling incoming food, or to cool a refrigerated storage room.
Although liquid nitrogen is capable of freezing food down to -196C, this is virtually never done, because it entails unnecessary cost and could even be damaging to some foods. The food is seldom frozen to a temperature below -45C, and quality results largely from the speed at which this temperature is reached. In the case of many fruit, vegetable, meat, and fish items this may require 1-3 min.
Much shrimp is liquid-nitrogen frozen in the IQF form. Typically, the shrimp enter the freezing tunnel at one end and a liquid-nitrogen spray is directed at the conveyor belt at the opposite end and the tunnel. The spray vaporizes and cold nitrogen gas is directed through the tunnel to meet the incoming shrimp in a countercurrent fashion. The incoming shrimp thus are first chilled y cold gas to about 0C before they reach the spray. Then they are passed under the liquid spray and are frozen to a surface temperature of about -185C. The shrimp are then moved through an equilibration zone where the cold surface and warmer core equilibrate to a uniform temperature of about -45C. The shrimp are then passed through a controlled water spray, where the stored refrigeration of the shrimp freezes a thin ice glaze protective against subsequent dehydration in storage. The shrimp then emerge from the glaze spray at about -30C for packaging and storage at about -23C. Generally, liquid-nitrogen freezing will produce less dehydration during freezing and less drip loss during thawing than other freezing methods. This can amount to as much as 5% of the weight of some foods.
Cryogenic freezing with carbon dioxide has taken two forms, in one, powdered dry ice, which sublimes at -79C, is mechanically mixed with the food to be frozen. In the other, liquid carbon dioxide under high pressure is sprayed onto the food surface. as pressure is released in the course of spraying, the liquid carbon dioxide becomes dry ice snow at -79C. There are applications where frozen food quality with this refrigerant is equal to that obtained with liquid-nitrogen freezing. In such cases, since a given weight of dry ice will absorb over twice as much heat in vaporizing as does liquid nitrogen, the use of carbon dioxide may have economic advantages over liquid-nitrogen freezing.
Packaging Considerations
The packaging of frozen foods imposes certain special requirements. Because of the tendency of water vapor to sublime from frozen food surfaces to colder surface in freezers and storage rooms, packaging materials for frozen foods should have a high degree of resistance to the permeation of water vapor. Most foods expand on freezing, some to the extent of 10% of their volume. Therefore, packages in which food is frozen should be strong and flexible. As with all foods that may be stored for months or years, packages should be protective against light and air. Because frozen foods generally will be thawed at time of use in their containers, packages should be liquid-tight to prevent leaking on thawing. Many packages and packaging materials such as cans, foils, waxed papers, plastic-coated paperboard, and plastic films are all satisfactory for frozen foods. Glass generally is not satisfactory due to breakage from expansion and from thermal shocks. Packaging will be considered in more detail in Chapter 21.
SOME ADDITIONAL DEVELOPMENTS
As in all industries, favorable economics encourage alternative methods of food processing. Freezing and frozen transportation and storage are highly energy intensive. Other methods of processing that may yield products equal or nearly equal in quality to those obtained by freezing have received increasing attention with rising energy costs. Currently, citrus juice concentrates are commonly frozen for high quality. However, juices and certain other foods may also be produced by HTST heating followed by aseptic packaging, as has been done in Europe for some years. Food irradiation, to be discussed in Chapter 11, also is capable of quality preservation of some products now routinely frozen. These and other methods can be less energy intensive and less costly than freezing and frozen storage but also have limitations. Frozen storage and display at the retail store is also expensive and retailers are looking for ways to reduce these costs.
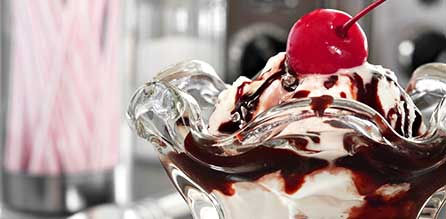
Comments