Fats and oils have been discussed in several previous chapters. The major portions of fats and oils are made up of fatty acid esters of glycerol. Edible fats and oils come from other plant and animal sources and have important functional and nutritional properties in foods. certain components of fats are required nutrients as well as carriers of the fat-soluble vitamins. Fats and oils can deteriorate in foods and are susceptible to oxidation and rancidity. They also have shortening, lubricating, emulsifying, and whipping properties, and high caloric value. The terms fat and oil only indicate whether the material is liquid or solid: fats that are liquid at room temperature are called oils.
EFFECT OF COMPOSITION ON FAT PROPERTIES
The structural formula of typical triglyceride molecule of a fat is shown in Fig. 16.1. In this case, three different fatty acids are esterified or connected to glycerol. There are numerous fatty acids. The structure of the fatty acids that are esterified to glycerol largely, determine the properties of fat, including whether they are solid or liquid at room temperature. It is well to review some of the more important properties these different fatty acids contribute to fats before considering the processing and utilization of fats and oils.
Short-chain fatty acids give softer fats of lower melting points than do long-chain fatty acids. Fatty acids can have areas of unsaturation within their molecules due to the absence of hydrogen atom at certain points. This is where double bonds occur in the fatty acids. In the triglyceride molecule of Fig. 16.1, all three fatty acids are of the same length –each contains 18 carbon atoms –but the degree of unsaturation of each is different. The top fatty acid, stearic acid, is fully saturate (i.e., it has no place where additional hydrogen atoms could be placed); the middle one, oleic acid, has one double bond and is missing four hydrogen atoms, so it is the most unsaturated.
The greater the degree of unsaturation in the fatty acids of fat molecules, the softer the fat is at a given temperature and the lower its melting point. When there is a considerable degree of unsaturation, the fat will be liquid at room temperature and will be called an oil.
By chemical means, hydrogen can be added to an oil to saturate its fatty acids, thereby converting it to a solid. This process is termed hydrogenation and commonly converts a vegetable oil to a solid shortening. Partial hydrogenation produces an intermediate degree of solidification. The degree of hydrogenation is important in determining the solid properties of foods containing fats.
Figure 16.1
Unsaturated fatty acids are highly reactive with oxygen at the points of unsaturation. Therefore, hydrogenation, which saturates fats, makes them more resistant to oxidation and more stable against oxidized flavor development.
Fatty acids, like many organic compounds, exhibit isomerism. Fatty acid isomers have the same numbers of carbon, hydrogen, and oxygen atoms but in different geometrical arrangements, which result in different chemical and physical properties. Fatty acids with the same empirical formula may have straight chains or branched chains, as with n-butyric acid and isobutyric acid. Fatty acids with one more double bonds can show two types of isomerism, namely, positional and geometric isomerism. Positional isomerism has to do with the position of the double bond or bonds along the carbon chain. Geometric isomerism is due to restricted rotation of two carbon atoms connected by a double bond. In this case, hydrogen atoms (or other groups) attached to the carbons of the double bond can be on the same side of the double bond (cis isomer) or on opposite sides of the double bond (trans isomer). Thus, oleic acid (cis form) can be converted to elaidic acid (trans form):
Most naturally occurring unsaturated fatty acids are in the cis form but may be changed to the trans form under certain conditions of processing. Isomers of a given molecular weight generally differ in melting point, solubility, stability, biological and nutritional properties, and in other ways, and these differences are imparted to the fats containing them.
Natural fats contain more than one kind of triglyceride molecule. A given fat will generally contain a mixture of triglyceride molecules differing in the lengths and in the degrees of unsaturation of their fatty acids (Table 16.1) and 16.2. Because of this, some molecules in the fat will be softer and some harder. The overall fat may be a liquid oil. Should the liquid fat be cooled, more of the fat molecules will solidify and they may form fat crystals, which separate from the liquid oil portion. This is one property that is used in separating fats into liquid and solid fractions. The liquid fraction will have a lower melting point and the solid fraction a higher melting point than the original mixture, and both fraction will be suitable for different food uses.
Figure 16.2 is a photomicrograph of a vegetable shortening with solidified fat crystals suspended in the liquid oil portion. The proportion of fat crystals and liquid oil depend on the melting points of the crystals, which will be low when the fatty acids in the crystals are of short length or are highly unsaturated. When this shortening is chilled, more crystals melt and the shortening becomes a liquid oil.
The fat and oil chemist and processor have as their raw material a versatile group of natural fats with different properties (Table 16.3). For example, the fat from cocoa, known as cocoa butter, is solid at temperature below 30C; on the other hand, the fat pressed from peanuts is liquid at room temperature. But the chemist and processor can also modify fats and oils of a given kind very readily by hydrogenation to make them stiffer, by temperature-controlled crystallization followed by separation into solid and liquid portions, and by still other means. The processor can then further blend various natural, hydrogenated, or crystallized fats into an endless number of mixtures and thereby further tailor special fats for the widest range of uses (Table 16.4).
SOURCES OF FAT AND OILS
Fats and oils may be of vegetable, animal, and marine origin. Examples of vegetable fats include the solid fat cocoa butter, and the liquid oils include corn oil, sunflower oil, soybean oil, cottonseed oil, peanut oil, olive oil, canola oil, and many more. Animal fats include lard from hogs, tallow from beef, and butterfat from milk. Fish oils include cod liver oil, oil from menhaden, and whale oil, although the whale is really a mammal.
Some of these fats and oils are chosen for food uses because of special flavor or physical attributes. For example, lard contribute a meaty flavor, olive oil has a distinctive flavor on salads, and butterfat has a buttery aroma and flavor. But in the production of shortenings, margarines, and frying fats, chemical and physical methods such as hydrogenation and crystallization are used to make many of the natural fats interchangeable with respect to texture and physical properties. Where permitted by law, flavors may be added to duplicate or even improve on the taste of a specific natural fat. Other fats and oils are chosen because they may have nutritional benefits. For example, oils from certain plants are highly unsaturated, which may have positive health benefits.

In the past, there has been spirited debate about the relative merits of different types of fat, and fierce competition among their makers. Prime examples of such controversies are butter versus margarine and lard shortening versus vegetable shortening. But in today’s market, economics and availability clearly dictate what fats will be used when the fats can be made interchangeable for a specific application by modern technology.
Because fats vary widely in price, and often can be made interchangeable or nearly, so, it is important that deception be prevented and consumers indeed get what they choose to purchase. Thus, there are federal standards of identity for foods, labeling requirements for no standardized foods, and analytical tests to distinguish between certain fats to prevent adulteration. Butter cannot be called butter if it contains any fat other than butterfat. This is also true of olive oil. Substitute cocoa and chocolate
Products must be appropriately labeled when they contain vegetable fats other than cocoa butter. But today, margarine, shortenings, frying fats and many other products are made from a wide variety of fat sources mostly of plant origin in accordance with availability and price.
FUNCTIONAL PROPERTIES OF FATS
Apart from flavor differences, when fats are used as shortenings, tenderizers, lubricants, frying media, whipping agents, and for other purposes, there are special requirements in each of these capacities.
*Solid Fat Index is related to the percentage of a fat that exists in crystalline form at a given temperature.
In the case of butter or table margarine, a plastic texture is required such that the butter or margarine will not become too hard in the refrigerator to spread, or too soft on a summer day so that it will run.
Oils used in salad oils should be clear and pourable. They should not contain high-melting point molecules that will solidify and crystallize when the salad oil is placed under refrigeration. Likewise, oils used in the preparation of mayonnaise should not form crystals when the mayonnaise is refrigerated, since these fat crystals could disrupt the state of emulsion and cause the mayonnaise to separate into fat and liquid phases.
Chocolate products should not melt at room temperature. They should be brittle and snap when bitten into, yet melt quickly in the mouth. Cocoa butter melts sharply above 30-36C and possesses thee properties. Chocolate substitute contain fats other than cocoa butter that may have been tailored to closely match these properties of cocoa butter. In the case of chocolate-type coatings for certain confections and crackers, however, it may not be the objective to match chocolate but rather to produce a coating with a higher melting point to prevent melting when handled.
Where the fat is to be whipped into a fat –air emulsion, as in the case of buttercream type icings, partially solidified shortenings generally produce a firmer whip than liquid oils. Also, where shortening is to be used in baked goods, a partially solidified fat generally functions better than most liquid oils, since the oils tend to separate and collect in pockets in the baked item.
Optimum fats for some of these and other application cannot always be obtained from nature. More generally, they must be fabricated by blending a variety of fats and oils that have undergone various degrees of modification. This is particularly true for fats and oils used in manufactured food produced with automatic equipment. Thus, the requirements of a shortening used in the high-speed continues bread-making process are different from those of a shortening for bread made by the batch method.
PRODUCTIOIN AND PROCESSING METHOD
There are only a few basic production methods to obtain fats and oils from animal, marine, and vegetable sources. These include rendering, pressure expelling, and solvent extraction, which are followed by various refining and modifying procedures.
Rendering
In the process of rendering meat scraps are heated in steam or water to cause the fat to melt. The melted fat then rises and water and remaining tissue settle bellow. The melted fat is then separated by skimming or centrifugation. Dry-heat rendering cooks the tissue under vacuum to remove moisture; wet rendering utilizes water and steam: and low-temperature rendering uses just enough heat to melt the fat. Low-temperature rendering can produce a fat of lighter color, but where meatier flavor is desired, higher-temperature rendering is used. Rendering also is used to obtain oil from whale blubber or fish tissue. In its simplest form, rendering can be carried out in a heated kettle; but large-capacity modern rendering plants are sophisticated and employ continuous rendering methods.
Pressing or Expelling
Various types of mechanical presses and expellers are used to squeeze oil from oilseeds. Seeds are usually first cooked slightly to partially break down the cell structure and to melt the fat for easier release of oil. The seeds also may be ground or cracked for the same purpose. The heat from cooking or grinding should not be excessive or it may darken the color of the oil. With some seeds (e.g., corn) only the germ portion of the seed is pressed to obtain oi, whereas with others, the entire seed is pressed. The expelled oil is usually further clarified of seed residues by being pumped through multiple filter cloths or by centrifugal clarification.
Solvent Extraction
It is common in large-scale operation to remove the oil from cracked seeds at low temperatures with a nontoxic fat solvent such as hexane. The solvent is percolated through the seeds, and after the oil is extracted, the solvent is distilled from the oil and recovered for reuse. Solvent extraction frequently will get more oil out of seeds than is possibly by pressing. Combined processes employ pressing to remove most of the oil followed by solvent extraction to recover final traces. The oil-free residual seed meal is then ground for animal feed. The stages in solvent extraction of soybeans are shown in Fig. 16.3
Degumming
Vegetable oils obtained by pressing or solvent extraction always contain fatlike substances, such as phospholipids or fat –protein complexes, which are gummy. When wetted with water, these materials become insoluble in the oil and settle out. This is one way to obtain the phospholipid lecithin.
Refining
Whereas water will settle much of the gummy material, use of alkali solution will settle additional minor impurities from the oil. These include free fatty acids, which combine with alkali to form soaps. These soaps can be removed by filtration or centrifugation. Treatment with alkali is known as refining.
Bleaching
Even after degumming and refining, the oil from seeds contains various plant pigments such as chlorophyll and carotene. These can be removed by passing heated oil over charcoal or various absorbent clays and earths. Animal fats generally can be bleached by heat alone.
Deodorization
Natural fats and oils from seeds, meat, and fish can contain low-molecular-weight odorous compounds. These are desirable in some products (e.g., olive oil, cocoa butter, lard, fresh butterfat, and chicken fat) and are not deliberately removed. But many other oils such as fish oils and several seed oils have disagreeable odors. These odors are removed by heat and vacuum or sometimes by adsorption onto activated charcoal. The heat is often supplied by injecting steam into the fat in low-pressure evaporators.
Figure 16.3
Hydrogenation
Hydrogenation to saturate fatty acid double bonds and thus change the viscosity of the fat is carried out by whipping deaerated hot oil with hydrogen gas plus a nickel catalyst in a closed vessel, known as a converter. When the desired degree of hardening of the fat is reached, the unreacted hydrogen gas is removed from the vessel by vacuum and the nickel catalyst is removed by filtration (Fig 16.4).
Hydrogenation not only saturates many double bonds but also produces trans isomers of various unsaturated fatty acids. When oleic acid (cis form) is converted to its trans isomer, elaidic acid, its melting point of about 14C is increased to about 44C. this increase in melting point also occurs when other cis isomers are changed to the trans form. Thus, hydrogenation increases the hardness of fats by this mechanism as well as by saturating double bonds; the extent to which each mechanism occurs can be influenced by temperature, pressure, time, and other hydrogenation variables. Hydrogenation also changes the nutritional properties of some fats. Polyunsaturated fatty acids not only become more saturated but the essential linoleic acid, and possibly other unsaturated fatty acids, lose biological activity when they are converted to the trans form. This is one of the reasons that diets should acquire part of their fat from un-hydrogenated sources.
Winterizing and Fractionation
As pointed out above, fats and oils are made up principally of a mixture of various triglycerides. The triglycerides containing more saturated fatty acids, and fatty acids of longer chain lengths, tend to crystallize out and settle from the mixture when an oil is chilled. Crystallization and settling in a refrigerated product such as salad oil can be prevented by cooling and removing the crystals before the final product is bottled. The precooling treatment to remove fat crystals is known as winterizing. It may be done by simply setting drums of oil in a cold room at a selected temperature that is lower than the product will later experience or by continuous processing through precisely controlled heat exchangers. One recent advance in fractionating the components of fats utilizes winterizing and the solubility of fats in solvents such as acetone. Here the fat is dissolved in the solvent and chilled to produce a crystalline fraction. The filtrate is recovered and then chilled to a lower temperature crystallize a second fraction, and so on.
Plasticizing and Tempering
The consistency and functional properties of more solid fats also are largely influenced by the state of crystallization. A given fat or oil can be modified by chilling and by agitation, both of which influence crystallization rat and crystal form. If a heated fat is allowed to cool slowly to a solidification temperature; it will have a different crystalline structure than if it is rapidly chilled o the same temperature; rapid chilling with agitation causes further difference. Controlled chilling with or without agitation to influence consistency and functional properties is referred to as plasticizing. It generally is done by pumping the melted fat or oil through a tubular scraped surface heat exchanger for super cooling, and then through a second chilled cylinder provided with a high-speed shaft containing rows of pins that alternate with pins on the cylinder wall to provide intensive agitation. For some applications, the crystallized fat also will be given a controlled degree of aeration by introducing measured amounts of air or nitrogen prior to chilling. A freshly plasticized fat undergoes further changes in consistency and functional properties upon standing. After a period of about 2-4 days at a temperature about 27C, these changes essentially cease. Holding a newly plasticized fat at a controlled temperature until its properties become stabilized is known as tempering. The chemical or physical basis for tempering become stabilized is known as tempering. The chemical or physical basis for tempering is poorly understood, but the improvements in tempered shortenings, such as an increased ability to emulsify water or air, are easily demonstrated. The ability of fat crystals to exist in different crystal forms in known as polymorphism.
Monoglyceride and Diglycerides Preparation
Glycerol esters containing only one or two fatty acid residues rather than the full three can be prepared from triglyceride plus about one-fifth their weight of additional glycerol. If the mixture is heated to about 200C in the presence of a sodium hydroxide catalyst, some of the fatty acid molecules disassociate from the triglycerides and react with free hydroxyl groups of the added glycerol. Since there is an excess of glycerol relative to total available fatty acid molecules, some of the hydroxyl groups of the glycerol must remain un-esterified. The reaction, known as glycerolizes, is carried out under an inert gas or under vacuum to prevent oxidation.
Monoglyceride and Diglycerides are both hydrophilic because of their free hydroxyl groups and hydrophobic due to their fatty acid residues. They are, thus, partially soluble in water and partially soluble in fat, which makes them excellent emulsifying agents. Monoglyceride and Diglycerides are commonly added to shortenings and many other food products for their emulsifying properties.
PRODUCTS MADE FROM FAT AND OILS
Food manufacturers use the many forms of fats and oils as ingredients in a wide variety of food products, or further process the fats and oils into manufactured products, a few of which are described in this section.
Butter
The raw material for butter is milk fat, usually in the form of cream, which is separated from the milk to contain about 30-35% fat. The cream is pasteurized at a somewhat higher temperature than for pasteurizing milk since the high fat content ha a slight protective effect on bacteria. Sometimes the cream is slightly acidic due to lactic acid from fermentation of the cream when it is separated on the farms and goes through several days of handling. When this is so, the cream is neutralized with a food-grade alkali prior to pasteurization.
Depending on the color of the cream, a vegetable coloring material (e.g., extract of annatto seed or carotene) may be added to deepen the yellow color. A measured amount of a lactic acid, di-acetyl producing bacterial culture also may be added to the cream to improve the butter flavor. The cream is now ready for churning.
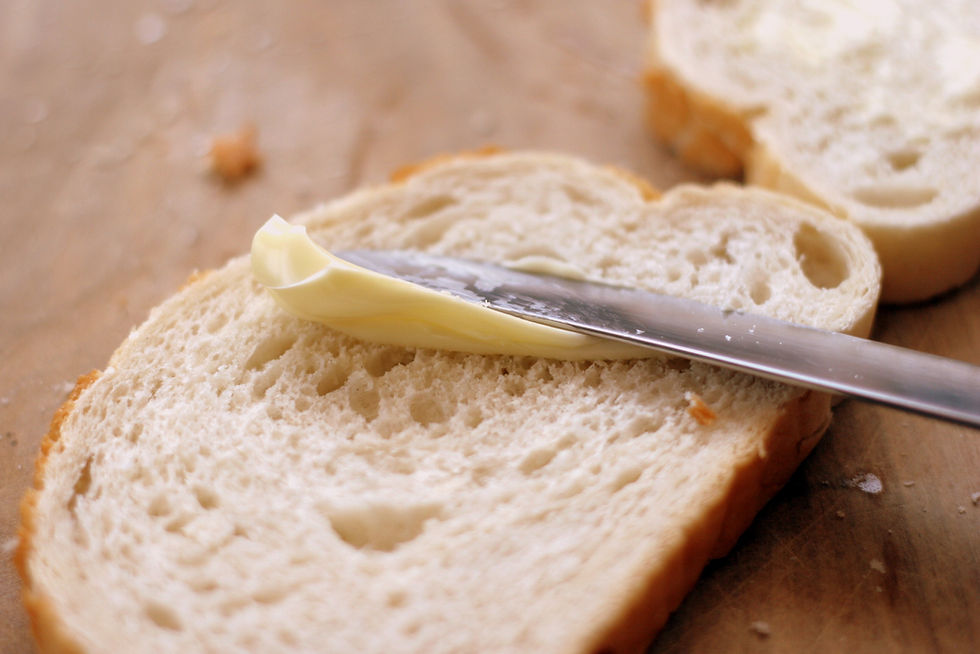
Churning, whether done as a batch operation or in a continuous process, is a mechanical agitation designed to reverse the natural emulsion in cream. The fat globules of cream are suspended in water such that the water is the continuous phase and the fat globules are the dispersed or discontinuous phase. Each fat globule is surrounded by a kind of phospholipid membrane, which contains lecithin, that helps to keep the globule emulsified or suspended in the water phase. The mechanical agitation of churning breaks this membrane like surface and causes globules to collide with one another. As a result, the globules clump together and from small butter granules; these grow in size and separate from the water phase of the cream. The resulting water phase or serum is known as buttermilk.
With the breaking of the emulsion, the butter granules mat together into a large mass of solid fat since the churn is operated at about 10C. At this point, the agitation or tumbling action of the churn is stopped and most of the buttermilk is drained from the churn. The state of the emulsion is now reversed. The mass of butterfat is the major component and it traps about 15% of buttermilk within it. The butterfat is now the continuous phase, and the remaining buttermilk, which is largely water with dissolved lactose, casein, and other milk solids, is suspended as droplets within the mass of fat. This condition results after about 40 min of batch churning.
The butter mass in a batch-type churn now appears as in Fig. 16.5. The mass next is washed with pure water by turning a hose into the churn to remove surface-adhering buttermilk. At this point, the wash water is drained and salt is added to the churn. A small amount of pure water also may be added to contribute the maximum amount of water permitted by law in the final butter. The churn again is closed and given further tumbling action to work the butter. The purpose of working is to uniformly disperse the salt and to subdivide the water droplets into smaller and smaller size. Subdivision of water droplets by proper working in the churn prevents these droplets from running together and causing leaky butter.
The salt, which is added at a level of about 2.5% of the final butter, contributes flavor and also acts as a preservative. All of the salt goes into solution in the water droplets, since the amount of water is only about 15% the salt concentration in the water is actually about seven times the 2.5% salt added. At this concentration, the salt is a strong preservative within the water droplets and largely prevents the growth of spoilage bacterial in these droplets.
The butter may now be packaged in large cartons or in smaller units with a machine known as a butter printer. In the latter case, the butter is loaded into the hopper of the machine from where it is mechanically extruded in the shape desired, cut to size, and wrapped.
Butter also may be made by high-speed continuous processes, but the basic principles cited for the batch churning operation apply to all. Typically, cream is pumped into cylindrical chilled churns that look somewhat like an ice cream freezer (Fig. 16.6). High-speed mixing within the cylinder forms the butter granules in a matter of seconds. The butter granules may be mechanically forced through perforated plates while the buttermilk simultaneously is drained from the cylinder. A salt solution is injected into an extension of the cylinder where the butter is further worked as it is extruded. The continuously extruded butter goes directly to automatic packaging equipment. The entire process can be operated under computer control (Fig. 16.7)
Figure 16.5
In the United States the legal standard for butter requires that the fat the entirely from butterfat and that the finished product contain not less than 80% butterfat by weight. Color and salt are optional.
Margarine
The term margarine applies to certain types of shortenings as well as table spreads. The consumption of table spreads in the United States has remained rather constant over the last 30 years; however, butter has declined in popularity while margarine has increased. Much of this is due to the lower price of margarine and nutritional differences of the two products.
In the United States, margarine is made largely from vegetable oils that have been hydrogenated or crystallized for the proper spreading texture. The vegetable oils may also be blended with lesser quantities of animal fats. The choice and blending of oils depend on seasonal availability, price, and nutritional differences like degree of saturation or unsaturation. Like butter, legal table margarine must contain on less than 80% fat. Since the oils are naturally almost 100% fat, water is added to produce the desired water-in-oil emulsion, which is physically quite the same as in butte. To the oil and water phases are added emulsifiers, salt, butter flavor, color, and permissible chemical preservatives such as sodium benzoate. Vitamins A and D also may be added.
In the manufacture of margarine two mixtures usually are made: one of the oil and all other fat-soluble ingredients, and the other of water and all water-soluble ingredients. These two mixtures are then emulsified in a vat with vigorous agitation, which distributes the water phase as small droplets throughout the continuous oil phase. The emulsion, which would quickly separate if not stiffened by chilling, is now quickly cooled. In modern continuous systems (Fig.16.8) this is done by pumping the emulsion through a series of heat exchangers which may have special agitators to further subdivide the water droplets throughout the fat as it stiffens. The emulsion is next passed through a chilled crystallizer to further solidify and plasticize the fat. Proper temperature control to develop optimum fat crystals is most important for producing the desired semi-plastic consistency. The semisolid margarine is continuously extruded and packaged as in continuous butter-making.
Whereas butter may contain only butterfat, most margarine contain only vegetable fats. However, some vegetable fat margarines are made to contain about 5-40% butter enhance flavor.
Butter and margarine are calorically concentrated and dense foods. recently, there has been a move to reduce the caloric content of these spreads. Two technologies have been used. He first is to increase the amount of air incorporated into the spread by whipping. This does not reduce the caloric content on a weight basis but does on a volume basis because the volume is increased by about 50%. The second method is to increase the amount of water incorporated in the spread by adding more plus better emulsifiers.
Shortening and Frying Oils
Margarine is often used in baking when its flavor, which results from the milk ingredients in it, is desired. Other bakery shortenings are made entirely of vegetable oils and are flavorless or may have butter flavors added to them. Others, particularly those favored for pie crusts, are made of lard. Many contain blends of vegetable and animal fats. Emulsified shortenings in addition contain monoglyceride, Diglycerides, and related compounds. These permit cake to be made with higher levels of water and sugar, and therefore to be moister and tender, than would be possible with un-emulsified shortenings.

Shortenings may be prepared to all degrees of stiffness including pour ability. Pourable shortenings are easily pumped and metered but are generally not equal to the plastic shortenings in bakery performance. One important function of s shortening is to hold air, whether beaten in a cake butter or creamed with other icing ingredients. This ability to hold air generally is increased by plastic consistency of the shortening. Further, following baking, plastic shortenings remain dispersed within baked goods, whereas liquid shortening have a tendency to leak and collect in pockets.
Two shortenings with the same initial hardness can have different softening properties as they are subjected to the same conditions of elevated temperature. This results from the different melting points of various triglycerides in each mixture. Such shortenings are said to have different plastic ranges; that is, they will remain semisolid over a wider or narrower temperature range. The solid Fat Index is a measure of the solidity of fats at various temperatures; it is related to the percentage of the fat that exists in crystalline form as distinct from melted oil at a given temperature. Solid Fat Index curves therefore correlate well with plastic ranges of fats and shortenings. Values of the Solid Fat Index are given in Tables 16.3 and 16.4.
A shortening that remains plastic over a wide range of temperature (long plastic range) is suited to most bakery operations. On the other hand, for frying, a long plastic range offers no advantages. On the contrary, shortenings with short plastic ranges and low melting points are preferred for frying operations. These properties minimize and low melting points are preferred for frying operations. These properties minimize greasiness from unmelted fat in the mouth when fried items are eaten.
Frying fats have additional requirements that are different from bakery shortenings. Whereas shortenings in baked goods seldom are exposed to temperatures much above the boiling point of water during baking, frying fats and oils are generally heated to about 160-190C in the frying kettle. Monoglyceride and Diglycerides decompose at such temperatures and produce smoke and, therefore, are not added to frying fats. Frying fats exposed to these high temperatures also must be given considerable stability against darkening, pyrolytic decomposition leading to gum formation, and oxidation. This is especially important in the case of industrial frying where fat is continuously filtered, reheated, and reused, as in the case of potato chip frying (Fig. 16.9). This generally calls for hydrogenation. Fats at high temperatures also have a tendency to foam in the frying kettle. This may be minimized by the incorporation of such materials as methyl silicones into the frying fats.
Figure 16.9
Frequently, somewhat conflicting attributes may be desired in fats and oils. For example, users want frying fats with maximum stability but also with low melting points to minimize greasiness in the mouth. Hydrogenation can contribute to the former but may aggravate the latter. On the other hand, fats with saturated shorter chain fatty acids can have both stability and relatively low melting points without hydrogenation. Although there is often more than one approach to solve such problems, the solution frequently must involve compromise influenced by the specific food application.
Mayonnaise and Salad Dressings
Standards of identity for mayonnaise in the United States require that it be made from at least 65% vegetable oil, 2.5% acetic or citric acid, and egg yolk. It may contain salt, natural sweeteners, spices, and various flavoring ingredients from natural sources. The acid is a microbial preservative. The egg yolk provides emulsification and a pale yellow color which may not be imitated or intensified. Commercial mayonnaise generally contains 77-82% of a winterized salad oil, 5.3-5.8% liquid egg yolk, 2.8-4.5% of a 10% acetic acid vinegar, small amounts of salt, sugar, and spices, and additional water to make 100%. In mayonnaise the oil phase is present in greater quantity than the water phase. Generally, the phase in greater quantity becomes the external or continuous phase when emulsions are made. In the case of mayonnaise, however, this is reversed to give an oil-in-water emulsion that has the characteristic viscosity, mouthfeel, and taste. Such an “unnatural” emulsion is difficult to prepare and tends to be relatively unstable. If the oil used in mayonnaise preparation is not properly winterized, then fat crystallization in a refrigerator will break the emulsion. Even when the oil is winterized, the mayonnaise emulsion quickly breaks upon freezing.
Mayonnaise is prepared commercially be batch and continuous methods, and there are many various with respect to order and rates of ingredient additions to the mixers. Two-stage mixing is commonly employed with high-speed turbine blades in the first stage followed by yet more severe of the oil into fine droplets in this second stage.

The second-stage mixer may possess close clearance whirling teeth as in a colloid mill. Mayonnaise may be whipped with small quantities of inert gas such nitrogen or carbon dioxide to produce a finished product with a specific gravity of 0.88-0.92. The gas commonly is pumped along with the emulsion into the second-stage mixer of enclosed design where the high shear mixing under pressure subdivides the gas into minute bubbles, which further contribute to “body” or firmness and texture. Mayonnaises with the same ingredient composition can vary greatly in their firmness, smoothness, sheen, spooning characteristics, and taste, and these properties are greatly influenced by the conditions of mixing. Mayonnaise is preserved against microbial spoilage by its acid content, but it is very sensitive to oxidative deterioration of flavor and should be refrigerated after jars are opened.
Salad dressings may be very similar to mayonnaise but generally contain less oil (35-50%) and contain a starch paste as a thickener. The egg yolk or other emulsifier, vinegar, and seasonings perform the same functions as in mayonnaise, and principles with respect to mixing and emulsion stability are similar except that special care must be given to starch cooking to develop the desire degree of thickening. For example, if the starch –water suspension is cooked together with the vinegar, acid hydrolysis tends to thin the starch paste. Therefore, it is preferred to add vinegar to the previously cooked starch paste, which is then blended with the oil, egg yolk, and other ingredients prior to final mixing –emulsification.
Pourable salad dressings, such as French dressing contain oil, vinegar, spices, and other ingredients. Pourable dressings may be fully emulsified or readily separate into oil and aqueous layers which are commonly shaken before use. Emulsifiers vary and include numerous gums as well as egg yolk. In the United States, French dressing composition is covered by a standard of identity that requires a minimum oil level of 35%, although higher levels of oil are common. Other pourable salad dressings can be quite variable in composition and flavoring ingredients. The separating types are well mixed but need not be emulsified prior to bottling.
FAT SUBSTITUETS
Because of the desire to reduce the caloric and fat content of the diet and to change the types of fat in the diet, many new fat substitutes have been or are being developed. These substitutes generally are designed to imitate the functionality of a particular fat in a food system at a reduced caloric content. They can generally be divided into two types: those which reduce caloric content by substituting a less calorically dense substance for the fat and those that behave like a fat but are not readily absorbed by the body and, thus, do not provide the same calories.
As pointed out earlier, fats in foods have several functions and some of those functions can be imitated by nonfat substances. For example, fats impart a smooth mouthfeel to many foods like chocolate and ice cream. Protein particles can be made very small, round, and hard. When suspended in water the small particles thicken and provide a smooth mouthfeel similar to that of fat. Because protein contain 100% of the caloric content of fat, the net effect is a reduction in calories. Such micro particular of proteins now serve as a popular fat substitute in such products as ice cream. Carbohydrates which can provide increased viscosity to water and thus mimic oils in such products as pourable salad dressings also reduce caloric content. One drawback to using proteins and carbohydrates as fat substitutes is that they do not withstand the high temperatures of frying or cooking.
The second type of fat substitutes, the sugar esters, are chemically similar to natural fats but are not absorbed and metabolized in the body. These substances will withstand the high temperature of frying but not contribute to caloric content of fried foods. Few of this type of fat substitute have been approved for foods at this time, but it is likely that they will be. The most well-known substance is Olestra® which is a chemical derivative of the common table sugar sucrose.
TESTS OF FAT AND OILS
Generally, fats and oils are tested to gain information related to performance in specific food applications, to measure degree of deterioration (such as oxidation or rancidity) as well as stability of the fat against such change, to check fat properties against purchase specifications, and to identify fats and oils against such change, to check fat properties sensation or adulteration. The important physical and chemical properties of fats and oils have been measured in various ways that have created a number of terms commonly associated with properties of fats.
Chemical Tests
The degree of unsaturation of the fatty acids in a fat or oil can be quantitatively expressed by the Iodine Value of the fat. Iodine Value refers to the number of grams of iodine absorbed by 100g of fa. Since the iodine reacts at the sites of unsaturation much as would hydrogen in hydrogenation, the higher the Iodine Value the greater the degree of unsaturation in the fat.
The degree of oxidation that has taken place in a fat or oil can be expressed in terms of Peroxide value. When the double bonds of unsaturated fats become oxidized, peroxide are among the oxidation products formed. Under standard conditions these peroxides can liberate iodine from potassium iodide added to the system. The amount of iodine liberated ins then a measure of peroxide content, which correlates with degree of oxidation already experienced by the fat and probable tendency of the fat to subsequent oxidation already experienced by the fat and probable tendency of the fat to subsequent oxidative rancidity. Oxidative rancidity results from the liberation of odorous products during breakdown of unsaturated fatty acids. These commonly include such compounds as aldehydes, ketones, and shorter-chain fatty acids. This is the type of fat deterioration that can often be prevented or minimized by the addition of chemical antioxidants, such as butylated hydroxyanisole (BHA) and butylated hydroxytoluene (BHT).
Fats also are degraded by the process of hydrolysis, which in the presence of moisture splits triglycerides into their basic components of glycerol and free fatty acids. The free fatty acids, especially if they are of short-chain length, cause off-odors and rancid flavors in fats and oils. This type of deterioration, referred to as hydrolytic rancidity, is to be distinguished from oxidative rancidity. Hydrolytic rancidity does not require oxygen to occur but is favored by the presence of moisture, high temperatures, and natural lipolytic enzymes. The term Acid Value refers to a measure of free fatty acids present in a fat. Acid Value is defined as the number of milligrams of potassium hydroxide necessary to neutralize 1 g of the fat or oil
The average molecular weight of the fatty acids in fat, which influences firmness of the fat as well as flavor and odor properties (low-molecular-weight fatty acids are more odorous), is another important property of fats and oils. The average molecular weight of the fatty acids in a fat is indicated by Saponification. Value, which is the number of milligrams of potassium hydroxide required to saponify (convert to soap) 1 g of fat. Since 1 g of fat must contain more fatty acids if they are of short-chain length or fewer fatty acids if they are of long-chain length, and the fatty acids react with the alkali to give the soap, it follows the Saponification Value increases and decreases inversely with average molecular weight.
These are but a few of the chemical tests that have been applied to fats and oils. Much that they reveal can be learned today more quickly by instrumental analytical methods such as gas chromatography and infrared absorption analysis, and many of the classical chemical tests have been largely replaced by these newer methods.
Physical Tests
The most important physical characteristic of fats is their consistency under different temperature conditions. Most fats and oils do not melt or solidify sharply at a given temperature. Rather, because fats and oils are mixtures of triglyceride molecules, each with its own melting point. Fats melt or solidify gradually over a temperature range.
There are various tests to indicate the beginning of melting of a fat or oil previously chilled to a specified temperature. In one type, the temperature at which the chilled cloudy fat within a capillary tube loses it cloudiness from melting of its solidified crystals is taken as the melting point. In another, the temperature at which chilled fat in a capillary tube softens just enough to slide within the tube is considered the melting point. A related type of test measures the temperature for a melted fat to go to the crystalline state y observing the point at which cloudiness of the fat is complete. This test can then be extended to determine the temperature at which the cloudy fat congeals.
The Solid Fat Index, mentioned earlier, is a measure of solidity of fats and is related to the percentage of the fat that is crystalline at specific temperatures. Crystallinity is measured by changes in volume that occur when fat crystals melt. The experimental method is referred to as dilatometry.
More sophisticated instrumental methods can be used to gain information ion the state of crystallinity in a fat. One of these is X-ray diffraction and is based on the ability of crystals to deflect an X-ray beam according to the spacing between molecules within the crystal. Another is differential scanning calorimetry, which measure transition energies as fat crystals form or melt and precise temperature at which these events occur.
Additional Tests
The consistency of semisolid fats can easily be measured by their resistance to penetration of a needle, ring, or cone. The response of fats and oils to frying temperatures can be indicated by such measurements as a smoke point, flash point, and fire point, which correspond to the temperatures at which these occurrences begin.
Many chemical and physical tests are highly useful in the identification and quality control of fats and oils as food ingredients (Table 16.5). Frequently, however, they may not correlate closely with optimum performance of a given fat in a specific application. For this reason, actual performance tests with the fat may be indispensable. Performance tests are essentially scaled-down versions of the actual application in which the fat will be used. Sometimes they impose still more severe conditions than will be encountered in the corresponding commercial operation to add conservatism to the evaluation.
Effective performance tests on fats and oils (or any other ingredients) will differ among plants manufacturing the same type products when there are differences in recipe, manufacturing procedures, or even stability requirements under varying distribution and marketing conditions. Thus, performance tests on shortening for cakes prepared in a small retail bakery would be different from the test to be performed in a large wholesale bakery employing automated high-speed operations and exposing its finished products to the variable associated with national distribution.
Comments