FOOD DETERIORATION AND ITS CONTROL
- FoodScience
- Jun 19, 2019
- 28 min read
Updated: Jun 21, 2019
All foods undergo varying degrees of deterioration during storage. Deterioration may include losses in organoleptic desirability, nutritional value, safety, and aesthetic appeal. Foods may change in color, texture, flavor, or another quality attribute as discussed in Chapter 6.
Food is subject to physical, chemical, and biological deterioration. The highly sensitive organic and inorganic compounds which make up food and the balance between these compounds, and the uniquely organized structured and dispersions that contribute to texture and consistency of unprocessed and manufactured products are affected by nearly every variable in the environment. Heat, cold, light and other radiation, oxygen, moisture, dryness, natural food enzymes, microorganisms and macro organisms, industrial contaminants, some foods in the presence of others, and time –all can adversely affect foods. This range of potentially destructive factors and the great diversity of natural and processed foods is why so many variations of several basic food preservation methods find application in modern food technology.
The rapidity with which foods spoil if proper measures are not taken is indicated in Table 7.1, which lists the useful storage life of typical plant and animal tissues at 21C. Meat, fish, and poultry can become inedible in less than a day at room temperature. This is also true for several fruits and leafy vegetables, raw milk, and many other products. Room temperature or field temperature can be much higher than 21C during much of the year in many parts of the world. Typically, slower rates of deterioration occur with foods that are low in moisture, high in sugar, salt, or acid, or modified in other ways. Nevertheless, even in our modern and efficient warehouses and supermarkets, shelf stable, refrigerated, and frozen foods undergo continuous change, necessitating stock rotation and product removal at definite intervals, which may be days, weeks, or month for such products as baked foods, soft cheese, and frozen specialties, respectively.
Rapid spoilage has significance in less developed areas as well as in the most highly advanced and organized societies. In less developed areas starvation because of spoilage has been known to occur in villages only 20-30 km from locations of a lush harvest. In highly advanced societies food production generally is centralized in areas where food can be most efficiently grown or processed. These areas in the United States can be half a continent or more distant from a population center where the food will be consumed. Unless the deteriorative factors are controlled, there would be no food for these population centers and, indeed, there could be no highly advanced society.
History has been made and wars won or lost over food deterioration and its control. Wars, and the need to provide food for armies in regions remote from areas of food production, have always focused attention on the problems of food deterioration, and this is still very true today.
It is interesting to note that some of the most important advances in preventing food deterioration have been made in time of war.
At the close of the eighteenth century France was at war and Napoleon’s armies were doing poorly on inadequate rations that frequently included spoiled meat and other unwholesome or unpalatable items. Similar problems, including elimination of scurvy, were facing the navy and merchant shipping. Prizes were offered as incentive to encourage development of useful methods of preserving food. From this came the discovery by Nicolas Appert that if food was sufficiently heated in a sealed container and the container not opened, the food would be preserved. Appert was awarded 12,000 francs and honored in 1809, and the world gained the art of food canning. It was not until the work of Pasteur some 50 years later, that growth of microorganisms was shown to be a major cause of food spoilage; this provided an explanation for Appert’s method of preservation.
One of the most important aspects of food science is an understanding of food deteriorative factors and their control. Commonly, various forms of preservation were developed long before an understanding of the principals involved were known; and many of the foods we prize today developed out of attempts to prevent deterioration and prolong storage life. One might not ordinarily think of butter as a means of preserving food, but long ago it was discovered that while milk deteriorated in a day or two, clumps of butter fat that formed when milk was agitated could be removed from the milk and would store for weeks or months. Similarly, cheese, smoked fish, dried fruits, and many fermented foods had their beginnings in attempts to slow down deteriorative processes.
SHELF LIFE AND DATING OF FOODS
The shelf life of a food is sometimes defined as the time it takes a product to decline to an unacceptable level. Of course, what is “acceptable” varies form one person to another. To some, the slightest odor in fish in unacceptable, whereas others may prefer fish which has developed considerable odor. In many cases, shelf life is taken as the time a product remains salable. In the final analysis, shelf life is a judgment that must be made by the food manufacturer or retailer. In many cases, the manufacturer must define a minimum acceptable quality (MAQ) for the product. The MAQ will depend on what degree of degradation will be allowed before the manufacturer no longer wants to sell the product.
The actual length of the shelf life of any given product will depend on a number of factors such as processing method, packaging, and storage conditions. For example, one cannot accurately say what the shelf life of fresh milk is without indicating the conditions. Milk held at room temperature ill have a different shelf life than milk held under good refrigeration. Of course, canned and processed milk stored at room temperature will outlast pasteurized milk held at refrigeration temperature.
It has become a widespread practice to add some form of dating system to retail packages of foods so that consumers may have some indication of the shelf life or freshness of the products they buy. Several types of code dates have emerged including the date of manufacture (“pack date”), the date the product was displayed (“display date”), the date by which the product should be sold (“sell by date”), the last date of maximal quality (“best used by date”).
Considerable effort has gone into predicting and monitoring shelf life in recent years. Methods of predicting are particularly useful for new products which do not have a history of distribution. Predictions are based on the mechanism of deterioration and the rate at which these deteriorations occur. One recent system for monitoring shelf life uses labels or tags on foods which respond to a combination of time and temperature to which the product has been exposed. These indicators are, hence, called “time –temperature” indicators and are based on the principle that both time and temperature are important in the spoilage of foods.
MAJOR CAUSES OF FOOD DETERIORATION
The major factors affecting food deterioration include the following:
1. Growth and activities of microorganisms, principally bacteria, yeast, and molds;
2. Activities of food enzymes and other chemical reactions within food itself;
3. Infestation by insects, parasites, and rodents;
4. Inappropriate temperature for a given food;
5. Either gain or loss of moisture;
6. Reaction with oxygen;
7. Light;
8. Physical stress of abuse;
9. Time
These factors can be divided into biological, chemical, and physical factors.
Often these factors do not operate in isolation. Bacteria, insects, and light, for example, can all be operating simultaneously to spoil food in the field or in a warehouse. Similarly, heat, moisture, and air simultaneously affect the multiplication and activities of bacteria, as well as the chemical activities of food enzymes. At any one time, many forms of deterioration may take place, depending on the food and environmental conditions. Effective preservation must eliminate or minimize all of these factors in a given food. For example, in the case of canned meats the meat is sealed in a metal can, which protects it from insects and rodents as well as from light, which could affect its color a possibly its nutritive value. The can also protects the meat from drying out. Vacuum is applied or the can is flushed with nitrogen to remove oxygen before sealing. The sealed can is then heated to kill microorganisms and to destroy meat enzymes. The processed cans are stored in a cool room and the length of time the cans are held in supermarkets and in our homes is limited. In this case the preservation method takes into account all of the major factors in food deterioration. It is well to considerer these factors individually.
Bacteria, Yeasts, and Molds
There are thousands of genera and species of microorganisms. Several hundred are associated in one way or another with food products. Not all cause dieses or food spoilage, and the growth of several types is actually desirable because they are used to make and preserve foods. The lactic acid-producing organisms used for alcohol production in making wine or beer, or for flavor production in other foods. However, except where these microorganisms are especially cultivated by selective inoculation or by controlled conditions for favor their growth over the growth of less desirable types, microorganism multiplication on or in foods frequently is the major cause of food deterioration.
Microorganisms capable of spoiling food are found everywhere –in the soil, water, and air; on the skins of cattle and the feathers of poultry; and in the intestines and other cavities of the animal body. They are found on the skins and peels of fruits and vegetables, and on the hulls of grain and the shells of nuts. They are found on food processing equipment that has not been sanitized, as well as on the hands, skin, and clothing of food-handling personnel.
A most important point, however, is that microorganisms generally are not found within healthy living tissue –such as within the flesh of animals, or the flesh or juice of plants. But they are always present to invade the flesh of animals, or the flesh or juice of plants. But they are always present to invade the flesh of plant or animals through a break in the sin, or if the skin is weakened by disease or death. In this case they may digest the skin and penetrate through it to the tissue below. In nearly all cases, the presence of spoilage organisms in foods is a result of contamination. Therefore, one of the major strategies in reducing food spoilage due to microorganisms is to reduce contamination by ensuring good sanitation practices.
Milk from a healthy cow is sterile as secreted, but becomes contaminated as it passes through the teat canals, which are body cavities. Milk becomes further contaminated from dirt on the cow’s hide, from the air, from dirty utensils and containers, and so on. Beef becomes contaminated when the animal is slaughtered and the protective skin is broken, especially during cutting. Fruits, vegetables, grains, and nuts become contaminated when the skins or shells are broken or weakened. This is also true of healthy eggs. The inside of a healthy egg is sterile, but the shell of the egg can be highly contaminated from passage through the chicken’s body cavity at time of laying.
Bacteria are single-celled organisms, many of which can be classified into one of three types based on the shape of individual cells (Fig. 7.1). These are the spherical shapes represented by several form of cocci, the rod shape of the bacilli and spiral forms possessed by the spirilla and vibrios. Many bacteria can move by means of whip like flagella. Some bacteria, yeast, and all molds produce spores, which are seed like packets and which under proper conditions can germinate into full-sized cells called “vegetable cells”. Spores are remarkably resistant to heat, chemicals, and other adverse conditions (Fig. 7.2). Bacterial spores are far more resistant than yeast or mold spores, and more resistant to most processing conditions than vegetative cells. Sterilization processes are designed specifically to inactivate these highly resistant bacterial spores.
All bacterial associated with foods are small. Most are of the order of one to a few micrometers (µm) in length and somewhat smaller than this in diameter. All bacterial can penetrate the smallest openings; many can pass through the natural pores of an egg shell once the natural bloom of the shell is own or washed away. Yeasts are somewhat larger, of the order of 20µm or so in individual cell length and about a third this size in diameter. Most yeasts are spherical or ellipsoidal.
Molds are still larger and more complex in structure (Fig. 7.3). They grow by a network of hair like fibers called mycelia and send up fruiting bodies that produce mold spores referred to as conidia. The blackness of bread mold and the blue-colored veins of blue cheese are due to conidia; beneath the fruiting heads, the hair like mycelia anchor the mold to the food. Mycelia are 1 µm or so in thickness and, like bacteria, can penetrate the smallest opening; in the case of a weakened fruit skin or egg shell, they can digest the skin and make their own route of penetration.
Bacteria, yeasts, and molds can attack virtually all food constituents. Some ferment sugars and hydrolyze starches and cellulose; other hydrolyze fats and produce rancidity; some digest proteins and produce putrid and ammonia like odors. Other types form acid and make food sour, produce gas and make food foamy, and form pigments and discolor foods. A few produce toxins and give rise to food poisoning. When food is contaminated under natural conditions, several types of organisms will be present together and contribute to a complex of simultaneous or sequential changes, which may include production of acid and gas, putrefaction, and discoloration.
Figure; 7.2 and 7.3
Bacteria, yeasts, and molds like warm, moist conditions. Most bacterial multiply best at temperatures between 16C and 38C; these are termed mesophilic. Some will grow at temperatures down to the freezing point of water and are called psychotropic or psychrophilic. Others will grow at temperatures as high as 82C, and we call these thermophilic. The spores of many bacterial will survive prolonged exposure to boiling water and then multiply when the temperature is lowered.
Some bacterial and all molds require oxygen for growth and are called aerobic. Other bacterial will not grow unless all free oxygen is absent and are designated anaerobic. Still others can grow under either aerobic or anaerobic conditions and are called facultative.
Most important is the tremendous rate at which bacterial and other microorganisms can multiply. Bacterial multiply by cell division. One cell becomes two, two become four, and so ion in exponential fashion. Under favorable conditions, bacterial can double their numbers every 30 min. under such conditions milk with an initial bacterial count of 100,000 or so per milliliter, which is not uncommon before pasteurization, if left standing at room temperature can reach a bacterial population of about 25 million in 24 h, and over 5 billion per milliliter in 96 h.
Food-Borne Disease
A special kind of food deterioration that may or may not alter a food’s organoleptic properties has to do with food-borne disease. Food-borne diseases are commonly classified as food infections or food intoxications. Whereas the distinction is sometimes imperfect, food infections involve microorganisms present in the food at time of consumption and cause disease upon ingestion. Where the toxin producer is a microorganism, it need not grow in the host to produce disease or even be present in food.
Staphylococcus aureus and Clostridium botulinum produce bacterial food poisoning by intoxication through the production of specific bacterial toxins. In fact, the toxin produced by C. botulinum is one of the most toxic substances known. Certain molds produce mycotoxins, the best known being the aflatoxins of Aspergillus flavus. Unlike the toxins of S. aureus and C. botulinum, which are highly toxic to man, aflatoxins may be more toxic to domestic animals than to man. However, their carcinogenic properties are cause for much concern since aflatoxins can be produced in a wide range of cereals, legumes, nuts, and other products allowed to become moldy. When such products occur in feeds, aflatoxins may subsequently be detected in the milk of animals consuming the feed and in cheese made from such milk.
Many bacteria can transmit food-borne infections capable of causing human disease. These include Clostridium perfringens, numerous members of the genus Salmonella, Shigella dysenteriae, Vibrio parahemolyticus, Streptococcus pyogens, Bacillus cereus, Campylobacter jejuni, and others. A number of viral infections also may be contracted by man through contaminated food that has not been adequately processed or handled, including infectious hepatitis, poliomyelitis, and various respiratory and intestinal disorders. Microorganisms which cause disease in humans are known as pathogenic or pathogens.
Scientists are still learning about food-borne diseases. Over the last decade or so, several bacteria that had not been thought to be transmitted by food and cause human disease have been found to do just that. Chief among these “newer” pathogens are Aero monas hydrophila, Yersinia enterocolitica, Listeria monocytogenses, Vibrio para haemolyticu and a particular type of Escherichia coli called 0157:H7. Of particular importance is the recent discovery that some food-borne pathogenic bacteria can multiply at temperatures as low as 3.3C(38F). This means that temperatures which have been considered good for refrigerated storage may not always keep food from becoming a hazard. Considerable research is ongoing into ways to further protect foods from these psychotropic pathogens. Some of the causes of food intoxications and infections are listed in Table 7.2, along with the types of foods usually involved and general comments on corrective practices.
Insects, Parasites, and Rodents
Insects are particularly destructive to cereal grains and to fruits and vegetables. Both in the field and in storage it has been estimated that insects destroy 5-10% of the U.S. grain crop annually. In some parts of the world the figure may be in excess of 50%. The insect problem is not just one of how much an insect can eat, but when insects eat, they damage the food and open it to bacterial, yeast, and mold infection, causing further destruction. A small insect hole in a melon, not so bad in itself, can result in the total decay of the melon from bacterial invasion. Insects have controlled in stored grain, fruit, and spices by the use of pesticides, inert atmospheres, or cold temperatures. The use of chemical pesticides on foods continues to raise questions of possible toxic effects and maximum safe levels, and there are currently active programs to increase plant resistance and other biological-based methods of insect control. Genetic engineering, for example, offers the possibility producing food plants which have the genes to make chemicals which are toxic to insects. When the pest eats the plant, the toxicant kills the insect. Many of these toxicants are only effective on insects and of little concern for humans or the environment.
Insects eggs may persist, or be laid, in food after processing, as for example in flour. An interesting method of destroying insect eggs is to throw the flour with high impact against a hard surface as in a centrifuge-type machine known as an Entoleter (Fig. 7.4). The impact destroys eggs. They remain in the flour, but no further insect multiplication results.
Commodities containing highly destructive insects are prohibited from import, port, and sometimes transport across state lines. In the United States, the Animal and Plant Health Inspection Service of the USDA is responsible for such regulations. Some states and many countries prohibit fresh fruits and vegetables from being imported in order to try and prevent spread of the insects.
An important food-borne parasite is the trichinosis nematode, Trichinella spiralis, which can enter hogs eating uncooked food wastes. The nematode penetrates the hog’s intestines and finds, its way into the pork. If the meat is not thoroughly cooked, the live worm can infect man. It also is possible to destroy the nematode by frozen storage. All pork and pork products are government inspected, but as a further safeguard they should be thoroughly cooked before being consumed. Fish may also harbor parasitic worms. One kind that invades saltwater types such as herring, cod, mackerel, and salmon belongs to the genus Anisakis. The worm, which can infect man, survives normal refrigeration but can be killed by heating or freezing. It has been a problem in Japan and the Netherland where eating raw fish is common. Another parasitic contaminant of foods that causes much distress is Entamoeba histolytica, responsible for amoebic dysentery. Cysts of this organism are transmitted in feces and may contaminate foods where raw human excrement is used as fertilizer for crops. Infected water and poor hygiene also spread the parasite.
The problems with rodents involve not only the quantity of food they may consume but also the filth with which they contaminate food. Rats live 2-3 years, may have three to five litters a year, and produce seven or eight young per litter. In parts of the world where they are poorly controlled, they contribute substantially to food shortages. Rodent urine and droppings harbor several kinds of disease-producing bacteria, and rats spread such human diseases as salmonellosis, leptospirosis, typhus fever, and plague.
Food Enzymes
Just as microorganisms possess enzymes that ferment, rancidity, and putrefy foods, healthy uninfected food plants and animals have their own enzyme complement, the activity of which largely survives harvest and slaughter. Cereal grains and seeds recovered after 60 years of storage still possessed the properties of respiration, germination, and growth –all enzyme controlled functions. Not only can enzyme activity persist throughout the entire useful life of many natural and manufactured foods, but this activity often is intensified after harvest and slaughter. This is because enzymatic reactions are delicately balanced in the normally functional living plant and animal; but the balance is upset when the animal is killed or the plant removed from the field. Thus, although pepsin helps digest protein in the animal intestine, it does not digest the intestine itself in the healthy living animal. However, when the body defenses cease on slaughter, pepsin does contribute to proteolysis of the organs containing it. A great many similar “runaway” enzymatic reactions can be found in plants.
Unless these enzymes are inactivated by heat, chemicals, radiation, or some other means, they continue to catalyze chemical reactions within foods after slaughter or harvest. Some of these reactions are highly desirable if not allowed to go too far –like continued ripening of tomatoes after they are picked, and natural tenderizing of beef on ageing. But ripening and tenderizing beyond an optimum point becomes deterioration; the weakened tissues fall subject to microbial infections and the deterioration reaches the point of rotting. This can happen in the field, supermarket, and home refrigerator, given sufficient time.
Heat and Cold
Quite beyond their effects on microorganisms, heat and cold can cause deterioration of food if not controlled. Within the moderate temperature range over which most food is handled, such as 10C-38C, the rate of chemical reaction is approximately doubled for every 10C rise in temperature. This includes the rates of many enzymatic as well as no enzymatic reactions. Excessive heat, of course, denatures proteins, breaks emulsions, dries out foods by removing moisture, and destroys vitamins.
Uncontrolled cold also will damage foods. If fruits and vegetables are allowed to freeze, they suffer discoloration, changes in texture, or cracked skins, leaving the food susceptible to attack by microorganisms. Freezing may also cause deterioration of liquid foods. If a container of milk is allowed to freeze, the emulsion will be broken and fat will separate. Freezing also will denature milk protein and cause it to curdle. Carefully controlled freezing on the other hand need not cause these defects.
Cold damage to foods does not necessarily require the extreme of freezing. Fruits and vegetables after harvest, like other living systems, have optimum temperature requirements. When held at refrigeration temperatures of about 4C, some are weakened or killed and deteriorative processes follow. This is termed “chill injury.” Table 7.4 gives a partial listing of chill injury of some fruits and vegetables held cold but above the freezing point. The deterioration includes off-color development, surface pitting, and various forms of decay. Bananas, lemons, squash, and tomatoes are examples of products that should be held at temperature no lower than about 10C for maximum quality retention.
Moisture and Dryness
AS already pointed out, excessive moisture pickup or loss causes substantial deteriorative changes in foods. Moisture is required for chemical reactions and for microorganism growth; excessive moisture can accelerate these types of deterioration. Excessive loss of moisture can also have detrimental effects particularly on appearance and texture. Moisture need not be present throughout the food to exert major effects.
Surface moisture resulting from slight changes in relative humidity can cause lumping and caking, as well as such surface defects as mottling, crystallization, and stickiness. The slightest amount of condensation on the surface of food can become a virtual pool for the multiplication of bacteria or the growth of mold.
This condensation need not come from the outside. In a moisture-barrier package, fruits or vegetables can give off moisture from respiration and transpiration. This moisture is then trapped within the package and can support the growth of destructive microorganisms. No respiring food in a moisture-barrier package headspace. This moisture can then condense on the surface of the food, particularly when storage temperature is permitted to decrease.
Oxygen
Whereas nitrogen, which makes up 79% of air, is inert from the perspective of foods, the 20% oxygen in the air is quite reactive and causes substantial deteriorative effects in many foods. Besides the destructive effects due to chemical oxidation of nutrients (especially vitamins A and C), food colors, favors, and other food constituents, oxygen is also essential for mold growth. All molds are aerobic and this is why they are found growing on the surface of foods and other substances or within cracks in these materials.
Atmospheric oxygen is excluded from foods by vacuum deaeration or inert gas purging in the course of processing, by vacuum-packaging or by flushing containers with nitrogen or carbon dioxide, and in some instances by adding to foods and containers oxygen scavengers, which promote removal of residual trace oxygen through chemical reaction.
In recent years, positive use has been made of the fact that certain gases influence the rate of deterioration of foods. Several products which are often stored under refrigeration have been packaged in containers in which the air has been removed and replaced with some other gas or gas mixture. Often this mixture is made up of all nitrogen or a mixture of nitrogen and carbon dioxide. These mixtures can reduce the rate of deterioration and substantially increase shelf life. This is known as modified atmosphere packaging and will be discussed in more detail in the chapter on packaging. the high-moisture-content pastas that are now sold are packaged in modified atmospheres that are devoid of oxygen which inhibits the growth of mold.
Light
As mentioned in Chapter 4, light destroys some vitamins, notably riboflavin, vitamins A, and vitamin C, and causes deterioration of many food colors. Milk in bottles exposed to the sun develops “sunlight” flavor due to light-induced fat oxidation and changes in the protein. Not all wavelengths making up natural or artificial light are equally absorbed by food constituents or are equally destructive. Surface discolorations of sausages and meat pigments are different under natural light and under fluorescent light that may be encountered in display cases. Sensitive foods often can be protected from light by opaque packaging or by incorporating compounds into glass and transparent films that screen out light, of specific wavelengths.
Time
After slaughter, harvest, or food manufacture there is a time when the quality of food is the highest. In many products this quality peak can be passed in the field in a day or two, or after harvest in a matter of hours. Fresh corn and peas are notable examples. The growth of microorganisms, destruction by insects, action of food enzymes, no enzymatic interaction of food constituents, loss of flavor volatiles, and the effects of heat, cold, moisture, oxygen, and light all progress with time. This is not to say that certain cheeses, sausages, wines, and other fermented foods, quality decreases with time and major goals of food-handling and preservation practices are to capture and maintain freshness. Adequate processing, packaging, and storage may prolong the shelf life of foods considerably but cannot extend it indefinitely. Eventually, the quality of any food product decreases. This is the reason for considerable interest in shelf life dating of processed foods, a subject that will be considered further in Chapter 24.
SOME PRINCIPLES OF FOOD PRESERVATION
In subsequent chapters several of the more important food preservation methods are treated individually. For now, we will enlarge somewhat on some of the general principles underlying control of food deterioration. These are the principles on which food preservation methods are largely based.
If foods are to be kept only for short periods of time, then there are two very simple rules:
Keep food alive as long as possible; kill the animal or plant just before it is to be used. A good example of this is keeping lobsters alive in a tank in a supermarket or restaurant –while alive and healthy, they do not seriously deteriorate. This is also practiced with fish, poultry, fruits, and vegetables where possible. Unfortunately, the possibilities are limited.
If the food must be killed, clean it, cover it, and cool it as quickly as possible. However, cleaning, covering, and cooking will only delay deterioration for a short time, for hours or perhaps at most for a few days. Microorganisms and natural food enzymes will not be destroyed or totally inactivated and so will take over very quickly.
For longer-term and practical preservation, as required for most of our food supply, further precautions are necessary. These are largely directed at inactivating or controlling microorganisms, enzymes, and reducing or eliminating chemical reaction which cause food spoilage.
CONTROL OF MICROORGANISMS
The most important means of controlling bacteria, yeasts, and molds are heat, cold, drying, aid, sugar, salt, smoke, air, chemicals, and radiation. Any one of these can also cause deterioration of foods and so it is a matter of balance: an amount of heat that will kill microorganisms but still leave the food acceptable, a level of a preservative chemical additive that will inhibit microbial growth but have minimum adverse effects on food components or human health. Indeed, he entire science of food preservation is one of compromise with respect to dosage or treatment.
Heat
Most bacteria, yeasts, and molds grow best in the temperature range of about 16-38C. thermophiles will grow in the range 66-82C. Most bacterial are killed in the range 82-93C, but many bacterial spores are not destroyed even by boiling water at 100C for 30 min. To ensure sterility, that is, total destruction of microorganisms including spores, a temperature of 121C (wet heat) must be maintained for 15 min or longer. This is generally done with steam under pressure, as in a laboratory autoclave or commercial retort. These and other temperature effects on microorganisms are listed in Table 7.5. Commercial pressure retorts of the kind used in the canning industry operate at temperatures and for time intervals adequate to destroy large numbers of highly resistant bacterial spores within the canned food. Sterility or “commercial sterility,” to be defined later, is essential because the food may be stored in the can for a year or longer.
Not all foods require the same amount of heat for sterilization. When foods are high in acid, such as tomatoes or orange juice, the killing power of heat is increased. A temperature of 93C for 15 min may be enough to gain sterility if sufficient acid is present. Safe temperature and times for different foods have been well established and are published in handbooks used by the canning industry.
Another fundamental point on the use of heat and other means of preservation: it is not always necessary to kill all microorganisms and produce a sterile product. It may be necessary to employ only sufficient heat to destroy disease producing organisms in the food. This is done in the case of pasteurized milk. Most of the bacterial and all of the disease-producing organisms that might be present in milk are destroyed by pasteurization at 63C for 30 min, but the milk is not sterile. Nor need it be, since it will be held in a refrigerator and consumed generally within a few days.
However, evaporated milk, which is intended to remain in a can at room temperature for months or even years must receive a greater heat treatment to ensure sterility or commercial sterility.
Cold
As started earlier, most bacteria, yeasts, and molds grow best in the temperature range 16-38C. psychrotrophs will grow down to 0C, growth is slow and becomes slower and becomes slower the colder it gets (see Table 7.5). When the water in food is completely frozen, there is no multiplication of microorganisms. But in some foods all of the water is not frozen until a temperature of -10C or lower is reached. This is because of dissolved sugars, salts, and other constituents, which depress the freezing point.
The slowing of microbial activity with decreased temperatures is the principal behind refrigeration and freezing preservation. However, it is important to note that although cold temperatures will slow microbial growth and activities and may kill a certain fraction of the bacterial population, cold, including severe freezing, cannot be depended on to kill all bacteria. This is illustrated by data in Table 7.6. An ice cream mix inoculated with typhoid bacterial still retained over 600,000 live bacterial per milliliter after 1 year in frozen storage. Not only do cold storage and freezing fail to sterilize foods but when the food is taken from cold storage and thawed, the surviving organisms often resume rapid growth since the food may have been damaged from the cold or frozen storage. As indicated above, recent studies have shown that some disease-causing bacterial can grow at refrigeration temperatures of 3.3C.
Drying
Microorganisms in a healthy growing state may contain in excess of 80% water. They get this water from the food in which they grow. If the water is removed from the food; water will also be removed from the bacterial cells and multiplication will stop. Partial drying will be less effective than total drying, although for some microorganisms, partial drying as in concentration may be quite sufficient to arrest bacterial growth and multiplication
Bacteria and yeasts generally require more moisture than molds, and so molds often will be found growing on semidry foods where bacterial and yeasts find conditions unfavorable. Examples are mold growing on stale bread and partially dried fruits.
Slight differences in relative humidity within the room in which food is held, or within a food package, can greatly affect the rate at which microorganisms multiply. Figure 7.5 depicts the number of organisms present on meat stored cold for 20 days at 75% and 95% relative humidity (RH). At each temperature, the higher humidity produced the higher population of microorganisms.
Since microorganisms can live in one part of a food that may differ in moisture and other physical and chemical conditions from the food just millimeters away, the “microenvironment” of the microorganisms must be considered. Thus, it is common to refer to water conditions in terms of specific activity. The term “water activity” is related to relative humidity. Relative humidity is defined as the ration of the partial pressure of water vapor in the air to the vapor pressure of pure water at the same temperature. Relative humidity refers to the atmosphere surrounding a material or solution. Water activity is a property of solutions and is the ratio of vapor pressure of the solution to the vapor pressure of pure water at the same temperature. Under equilibrium conditions, water activity equals RH/100. Moisture requirements of microorganisms really means water activity in the immediate environment, whether it be in solution, in a particle of food, or at a surface in contact with the atmosphere. At the usual temperature permitting microbial growth, most bacterial require a water activity in the range 0.90-1.00. Some years and molds grow slowly at a water activity as low as 0.65. More will be said about water activity in chapter 10.
As stated previously, food is dried either partially or completely for several reasons. One of the most important is to preserve it against microbial spoilage. However, partial or complete drying of food does not kill all microorganisms. It may actually preserve microorganisms as it preserves the food, and dried food usually is far from sterile. Although bacterial may not grow in the dried food, when the food is remoistened or reconstituted, bacterial growth may resume.
Acid
In sufficient strength acid modifies bacterial proteins as it denatures food proteins and so microorganisms are sensitive to acid. Some are much more sensitive than others, and the acid produced by one organism during fermentation often will inhibit another type of organism. This is one of the principles of controlled fermentation as a means of preserving foods against growth of proteolytic and other type spoilage organisms.
Acid may be produced in foods by adding acid-producing bacterial cultures, or acids may be added directly to foods; examples are citric acid and phosphoric acid added to soft drinks. Foods such as tomatoes, citrus juices, and apples contain natural acidity with varying degrees of preservative power. Much of this is due directly to the hydrogen ion concentration (expressed in terms of Ph.), but two, acids producing the same ph. may not be equally preservative since the anions of certain acids also exert an effect. The degree of acidity tolerable in foods from the standpoint of palatability is never sufficient to ensure food sterility.
As mentioned earlier, acid combined with heat makes the heat more destructive to microorganisms. This is seen in Table 7.7 with respect to requirements for destruction of the spores of the anaerobic toxin-producing bacterium, C. botulinum,
Sugar and Salt
Fruits are preserved by placing them in a sugar syrup, and certain meat products are preserved by placing them in a salt brine. How does this work? Bacteria, yeasts, and molds are contained by cell membranes. These membranes allow water to pass in and out of the cells. Active microorganisms may contain in excess of 80% water. When bacteria, yeasts, or molds are placed in a heavy sugar syrup or salt brine, water in the cells moves out through the membrane and into the concentrated syrup or brine. This is the process of osmosis; in this case water moves from the cell containing 80% water into the syrup or brine, which may contain only 30% or 40% water.
The tendency to equalize water concentration inside and outside the cell in this case causes a partial dehydration of the cell, referred to as plasmolysis, which interferes with microorganism multiplication. Quite the opposite can be accomplished by placing microorganisms in distilled water. In this case water enters the cells and causes them to burst. This process, known as plasmoptysis, rarely occurs in food products. All of this is closely related to the water activity of solution and foods. Solutions high in solute concentration have a high osmotic pressure and a low water activity. Dilute solutions are low in osmotic pressure and have a high water activity. The quantitative contribution of a specific solute to osmotic pressure and water activity depends on the solute’s molecular weight and the number of ions it produces in solution. An equal weight of a low-molecular-weight solute has a greater effect on increasing a solution’s osmotic pressure and decreasing its water activity than the same weight of a high-molecular-weight solute.
Different organisms have various degrees of tolerance to osmosis and to sugar and salt. Yeasts and molds are more tolerant than most bacteria. This is why yeasts and molds often are found growing on high sugar or salt products; for example, fruit jam or bacon, where bacterial are inhibited.
Smoke
As with most preservative methods, smoke was used long before the reasons for its effectiveness were understood. In preserving foods such as meats and fish with smoke, the preservative action generally comes from a combination of factors. Smoke contains preservative chemicals such as small amount of formaldehyde and other materials from the burning of wood. In addition, smoke generally is associated with heat which helps kill microorganisms. This heat also tends to dry out the food, which further contributes to preservation. Smoking over a fire may be quite effective in preserving certain foods; on the other hand, today smoke may be added merely to flavor food, that is, without heat from burning. In this case the smoke may be a very poor preservative. In meat products such as those illustrated in Fig. 7.6, smoke combined with other preservatives is used more for its flavor than for its preservative action.
Atmosphere composition
The different requirements of microorganisms for oxygen and some of the means for removing air and oxygen already have been cited. To control organisms that require it, air is removed; for organisms that cannot tolerate it, air is provided. It is often fairly easy to exclude air from aerobes such as molds. Wax coating of cheeses or oxygen impermeable skin-tight plastic films can be quite effective. Controlling strict anaerobes by providing air can be more difficult and dangerous, especially with large food pieces. This is because the center of the food piece may remain anaerobic even if air is left in the headspace of a package. Further, some organisms consume oxygen and thus convert a formerly aerobic microenvironment into an anaerobic one favorable to other organisms. Thus, in preserving food against C. botulinum which is a strict anaerobe, measures in addition to inclusion of air are essential.
Chemicals
Many chemicals will kill or inhibit the growth of microorganisms, but most of these are not permitted in foods. A few that are permitted, in prescribed low levels in certain foods, include sodium benzoate, ascorbic acid, sodium and calcium propionate, ethyl format, and sulfur dioxide.
The use of chemicals to control microorganisms, and for any other purpose in foods, is part of the broader subject of food additives, which will be dealt with in a subsequent chapter. At the present time the Food Drug, and Cosmetic Act, administered by the Food and Drug Administration, regulates what chemicals may be used in foods, and the conditions of their use. New chemicals to be approved must undergo rigorous toxicological testing, and the burden of proof of safety resides with the producer or user of the chemicals. The FDA also retains the right to reverse former decisions and prohibit use of approved chemicals if new knowledge relative to safety warrants such action.
These chemicals can inhibit the growth of specific organisms. For example, ascorbic acid is effective at inhibiting the growth of many molds. Thus, the surface of cheese may be treated with sorbic acid or cottage cheese may have sorbic acid mixed directly in the product.
Radiation
Microorganisms are inactivated to various degrees’ y different kinds of radiation. X-rays, microwaves, ultraviolet light, and ionizing radiations are each a type of electromagnetic radiation, differing in wavelength and energy, that have been used to preserve food. The effectiveness of each type differs and each imparts different changes in food. For all types of radiation, the doses required to effectively sterilize most foods, and inactivate their natural enzymes, are generally excessive or borderline from the standpoint of food quality; and all may cause flavor, color, texture, or nutritional defects. Doses less than sterilizing appear more generally useful to extend storage life. These sub sterilization doses can inactivate enzymes responsible for initiating vegetable sprouting or can kill human disease-causing bacteria and insects.
Today, foods usually are irradiated with ionizing radiation, obtained from radioactive isotopes or electron accelerators. There is no significant temperature rise from this as spices, vegetables and fruits, pork, and poultry have been approved for irradiation, pasteurization at specific doses in the United States and other countries. Safety of irradiated food in the United States comes under the jurisdiction of the FDA. Food irradiation, which is receiving renewed interested in the United States, will be considered in more detail in Food irradiation and OHM processing of food.
CONTROL OF ENZYMES AND OTHER FACTORS
Most of what has been said in this chapter regarding control of deteriorative factors has dealt specifically with microorganisms. This emphasis is justified because by far the greatest losses to the food supply are due to microorganisms. Future, preservation of food against deterioration from inherent food enzymes, probably the second greatest cause of spoilage, follows many of the same principles and methods that apply to preservation against microbial deterioration.
Just as microorganisms are controlled with heat, cold, drying, certain chemicals, and radiation, these are the principal means used to control and inactivate damaging inherent food enzymes. Indeed, when foods are sterilized or pasteurized to inactivate microorganisms, natural food enzymes are simultaneously partially or completely destroyed. Likewise, when cold is employed to slow down microbial activity, it can also retard the activity of natural food enzymes.
It is important to recognize that some natural food enzymes may be more resistant to the effects of heat, cold, drying, radiation, and other means of preservation than microorganisms. Thus, a heat or a radiation, treatment may effectively destroy bacteria but leave damaging food enzymes intact to carry on food deterioration. Although a few broad generalizations can be made, specific condition for preservation must be selected in accordance with the unique spoilage patterns of individual food. Nevertheless, in two areas of preservation especially, irradiation and freezing, conditions that destroy or retard microorganisms’ activity frequently leave enzymes active.
As for preservation against such other factors as moisture, dryness, air, and light, protective packaging is the major means employed to retard losses. Insects and rodents are also largely controlled by protective packaging combined with a high degree of sanitation.
Many harmful substances can enter the food supply for which the control measures discussed here are of little value. Among these are industrial pollutants and pesticides. For example, radioactive nucleotides were found in foods in Europe as a result of the releases at the Russian nuclear power plant at Chernobyl. Many of these substances are not readily degraded, and if permitted to contaminate the environment, they find their way into foods by numerous routes (Fig. 7.7). These pose especially difficult problems for the Environmental Protection Agency and FDA. These agencies have developed elaborate quarantine and other plans in the event of disasters, but greater protection resides in strict preventative regulation and surveillance.
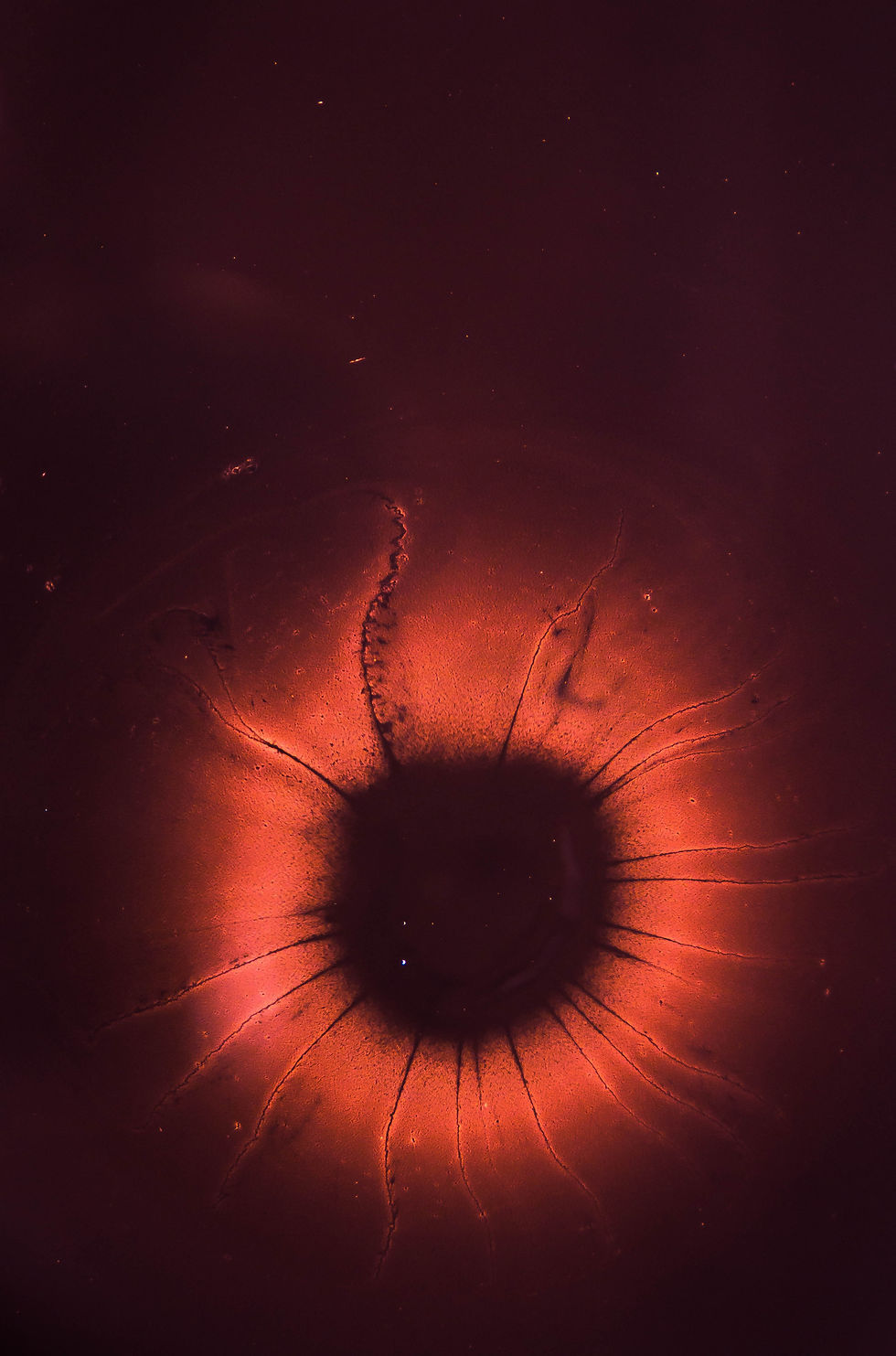
Comentarios