Of the various means of preserving foods, the use of eat finds very wide application. The simple acts of cooking, frying, broiling, or otherwise heating foods prior to consumption are forms of food preservation. In addition to making foods more tender and palatable, cooking destroys a large proportion of the microorganisms and natural enzymes in foods; thus, cooked foods generally can be held longer than uncooked foods. However, cooking generally does not sterilize a product, so even if it is protected from recontamination, food will spoil in a comparatively short period of time. This time is prolonged if the cooked foods are refrigerated. These are common household practices.
Another feature of cooking is that is usually the last treatment food receives prior to be consumed. The toxin that can be formed by Clostridium botulinum is destroyed by a 10-min exposure to moist heat at 100C. Properly processed commercial foods will be free of this toxin. Cooking provides a final measure of protection in those unfortunate cases where a processing error does occur, or a faulty food container becomes contaminated. However, heat preservation of food generally refers to controlled processes that are performed commercially, such as balancing, pasteurizing, and canning.
DIGREES OF PRESERVATION
It is important to recognize that there are various degrees of preservation by heating, and that commercial heat-preserved foods are not truly sterile. A few terms must be defined and understood.
Sterilization
Sterilization refers to the complete destruction of microorganisms. Because of the resistance of certain bacterial spores to heat, this frequently requires a treatment. If a can of food is to be sterilized, then immersing it into a 121C of wet heat for 15 min or its equivalent. It also means that every particle of the food must receive this heat treatment. If a can of food is to be sterilized, then immersing it into a 121C pressure cooker or retort for 15 min will not be sufficient because of the relatively slow rate of heat transfer through the food into the can. Depending on the size of the can, the effective time to achieve true sterility may be several hours. During this time there can be many changes in the food which reduce its quality. Fortunately, many foods need not be completely sterile to be safe and have keeping quality.
Commercially Sterile
The term commercially sterile or the word “sterile” (in quotes), sometimes seen in the literature, means that degree of sterilization at which all pathogenic and toxin forming organisms have been destroyed, as well as all other types of organisms which if present could grow in the product and produce spoilage under normal handling and storage condition. Commercially sterilized foods may contain a small number of heat resistant bacterial spores, but these will not normally multiply in the food supply. However, if they were isolated from the food and given special environmental conditions, they could be shown to be alive.
Most canned and bottled food products are commercially sterile and have a shelf life of 2 years or more. Even after longer periods, so-called deterioration is generally due to texture or flavor changes rather than to microorganisms’ growth.
Pasteurization
Pasteurization involves a comparatively low order of heat treatment, generally at temperature below the boiling point of water. Pasteurization treatments, depending on the food, have two different primary objectives. In the case of some products, notably milk and liquid eggs, pasteurization processes are specifically designed to destroy pathogenic organisms that may be associated with the food and could have public health significance. The second, more general, objective of pasteurization is to extend product shelf life from a microbial and enzymatic point of view. This is the objective when beer, wine, fruit juices and certain other foods are pasteurized. In the latter case, these foods would not be expected to be a source of pathogens, or would be protected by some other means of control. Pasteurized products will still contain many living organisms capable of growth of the order of thousands per milliliter or per gram –limiting the storage life compared to commercially sterile products. Pasteurization frequently is combined with another means of preservation, and many pasteurized foods must be stored under refrigeration. Pasteurized milk may be kept stored in a home refrigerator for a week or longer developing significant off-flavors. Stored at room temperature, however, pasteurized milk may spoil in a day or two. Pasteurization is not limited to liquid foods. A newer application is the steaming of oysters in the shell to reduce bacterial counts.
Blanching
Blanching is a kind of pasteurization generally applied to fruits and vegetables primarily to inactivate natural food enzymes. This is common practice when such products are to be frozen, since frozen storage in itself would not completely arrest enzyme activity. Blanching, depending on its severity, also will destroy some microorganisms, as pasteurization will inactivate some enzymes.
SELECTIN HEAT TREATMENST
Heat sufficient to destroy microorganisms and food enzymes also generally affect other properties of foods adversely. The mildest heat treatments that guarantee free-down from pathogens and toxins and produce the desired storage life will be the heat treatments of choice. How then do processors choose the optimal heat treatment for a particular food? To select a safe heat-preservation treatment, the following must be know:
Time-temperature combination required to inactive the most heat-resistant pathogens and spoilage organisms in a particular food.
Heat-preservation characteristics in a particular food, including the can or container of choice if it is packaged.
Processors must provide the heat treatment which will ensure that the remotest particle of food in a batch or within a container will receive sufficient heat, for sufficient time, to inactivate both the most resistant pathogen and the most resistant spoilage organisms if they are to achieve sterility or commercial sterility, and to inactivate the most heat-resistant pathogen if pasteurization for public health purposes is the goal. Different foods will support growth of different pathogens and different spoilage organisms, and so the targets will vary depending on the food to be heated.
HEAT RESISTANCE OF MICROORGANISMS
The most heat-resistant pathogen found in foods, especially those that are canned and held under anaerobic conditions, is Clostridium, botulinum. However, there are nonpathogenic spore-forming spoilage bacteria, such as Putrefactive Anaerobe 3679 (PA 3679) and Bacillus stearothermophilus (FS 1518), which are even more heat resistant than C. botulinum. If a heat treatment inactivates these spoilage organisms, C. botulinum and all other pathogens in the food also will be destroyed.
Margin of Safety
Data from thermal death curves can be plotted in various ways. In Fig. 8.4, data are plotted to show the heat resistance of bacterial spore suspensions as a function of initial spore concentration. Regardless of the temperature chosen, the greater the number of microorganisms or spores, the greater the heat treatment that will be required to destroy them.
We generally do not know how many organisms are present in food be commercially sterilized, or indeed which specific types of organism are present. To provide a substantial margin or safety in low acid foods, we may assume that a highly heat resistant spore former such as C. botulinum is present and that its population is large. From its thermal death rate curve established in the same food (or established in medium giving no greater protection against heat destruction), we may take its D value at the temperature we choose to employ and heat for a time such that every particle of food in the can is exposed to this temperature for a period equal to 12 D values. This is sufficient to decrease any population of C. botulinum through 12 log cycles. Since even highly spoiled food rarely supports a bacterial population greater than a billion organisms per can, 12 D values will bring the microbial population of the can to condition of sterility. Had a great number of such cans originally contained 1 billion C. botulinum organisms, then statistically, after a 12 D heat treatment, only 1 can in 1000 would be expected to still harbor I living organism or spore; the other 999 cans would be sterile. Had the food contained 1 million organisms per can before heating (which is still unusually high), then the same 12 D heat treatment would be expected to render 999,999 cans out of 1 million sterile. Since the 12 D heat treatment was based also on destroying C. botulinum, it would be still more effective against less heat-resistant spore formers and other far less heat-resistant non spore formation pathogens or spoilage organisms that might be present. When organisms still more heat resistant than C. botulinum are chosen as targets for destruction, then a heat treatment of less than 12 D values against these may be adequate. Thus, against Putrefactive Anaerobe 3679 (PA 3679) or Bacillus stearothermophilus (FS 1518) in low acid foods, a 5 D heat treatment is considered essentially equal to a 12 D value against C. botulinum and quite sufficient to eliminate microbial spoilage and render the product pathogen free. However, at present there is no general agreement on what theoretical number of survivors of different microorganisms is best for process calculations.
These heat treatments, which are commonly employed in the canning industry for low-acid foods, would be excessive and unnecessary for acid foods. Acid foods are currently defined as foods having a ph of 4.6 or less. Low-acid foods are those foods with a ph greater than 4.6. Table 8.1 gives the ph values for a number of foods, common spoilage agents associated with these foods, and an indication of the degree of heat processing required for their treatment. With many acid foods, temperatures at or below 100 C for a few minutes constitute adequate heat treatment.
Certain spices and food chemical is also combine with heat in killing microorganisms and so reduce the heat treatment that must be used. Still another factor in permitting lesser heat treatments to be used with acid foods is the sensitivity of C. botulinum to acid. Clostridium botulinum will not grow in foods at ph 4.6 or below. Therefore, such foods even if unheated would not constitute a health hazard from the standpoint of this heat-resistant organism.
Thermal Death Curves
Bacteria are killed by heat at a rate that is very nearly proportional to the number present in the system being heated. This is referred to as a logarithmic order of death, which means that under constant thermal conditions the same percentage of the bacterial population will be destroyed in a given time interval, regardless of the size of the surviving population. In other world, if a given temperature kills 90% of the population in the first minute of heating, 90% of the remaining population will be killed in the second minute, 90% of what is left will be killed in the third minute, and so on. This principle is illustrated in Fig. 8.1. The logarithmic order of death also applies to bacterial spores, but the slope of the death curve will differ from that of vegetative cells, reflecting the greater heat resistance of spores.
Figure 8.1 also illustrates the concept of the “D value” which is defined as the time in minutes at a specified temperature required to destroy 90% of the organisms in a population. Thus, the D value, or decimal reduction time, decreases the surviving population by one log cycle. If a quantity of food in a can contained one million organisms and it received heat for a time equal to four D values, then it would still contain 100 surviving organisms. If there were 100 such cans in a retort initially and the retort provided heat for a period equivalent to 7 D values, then it would be expected that the 100 cans with a total initial bacterial population of 100 million organisms would still contain 10 surviving organisms. Statistically, these 10 organisms should be distributed among the cans. Obviously, no container can have a fraction of an organism although the 100 cans will average 0.1 organism per can. In this case, 10 of the cans probably will have one organism each and could possibly ultimately spoil, while 90 of the cans would be sterile.
Figure: 8.1
Figure 8.1 is one kind of thermal death rate curve. It provides data on the rate of destruction of a specific organism in a specific medium or food at a specific temperature. From thermal death rate curves determined at different temperatures, a thermal death time curve (Fig. 8.2) can be constructed. A thermal death time curve for a specific organism in a specific medium or food provides data on the destruction times for a defined population of that organism at different temperatures.
Figure 8.2 illustrates two terms to characterize thermal death time curves. These are the “z value” and the “F value”. The z value is the number of degrees required for a specific thermal death time curve to pass through one log cycle (change by a factor of 10). It is also the negative slope index of the thermal death time curve. Different organisms in a given food will have different z values, which characterize resistance of the population to changing temperature. Similarly, a given organism will have different z values in different foods. The F value is defined as the number of minutes at a specific temperature required to destroy a specified number of organisms having a specific z value. Thus, the F value is a measure of the capacity of a heat treatment to sterilize.
Since F value represent the number of minutes to diminish a population with a specific z value at a specific temperature, and z values as well as temperatures vary, it is convenient to designate a reference F value. Such a reference is the F value, which equal the number of minutes at 121 C (250F) required to destroy a specified number of organisms whose z value is 10C (18F). If such a population is destroyed in 6 min at 121 C, then the heat treatment was equal to an F of 6. Other temperatures for different times can have the same lethality as this heat treatment. If they do, then they also can be described as having an F value of 6. If they have less lethality, they have an F value of less than 6 and vice versa. The F value of a heat treatment is thus a measure of its lethality, and the F value is also known as the “sterilization value” of the heat treatment. F is a common term in the canning industry and other areas utilizing heat processes. Not only do different amounts of heating provide different F values but the F requirements of various foods differ and are a measure of the ease or difficulty with which these foods can be heat-sterilized.
Figure: 8.2
Thermal death time curves have been carefully determined for many important pathogens and food spoilage organisms. Two such curves for Putrefactive Anaerobe 3679 and Bacillus stearothermophilus (FS 1518) are shown in Fig. 8.3. They tell us how long it takes to kill these organisms (under defined conditions) at a chosen temperature. Thus, for example, it would take about 60 min at 104 C (220 F) to kill a specified number of spores of PA 3679. On the other hand, at a temperature of 121 C (250F), these spores are killed in little over 1 min.
Figure: 8.3
The conditions that must be defined to make a thermal death curve meaningful and applicable to food processing are many. The requirement for a greater heat treatment the larger the initial microbial population is inherent in the logarithmic order by which bacterial die. In addition, the sensitivities of microorganisms to heat (and therefore the characteristics of the thermal death curve) are markedly affected by the composition of the food in which the heating is done. It already has been pointed out that acid increases the killing power of heat. As will be enlarged upon shortly, many food constituents have an opposite effect on heat sensitivity of microorganisms and protect them against heat. Thus, a thermal death curve established in a synthetic medium or in a given food generally is not applicable to a different food, and thermal death curves, to be valid, should be established in the specific food for which a heat process is being designed.
HEAT TRANSEER
Even after the time and temperature required to destroy target organisms are known from thermal death curves and a sufficient margin of safety has been calculated, a problem remains: how to ensure that every particle of food (within the container if the food is canned) receives the required heat treatment. This becomes a problem of heat transfer, that is, heat penetration into and throughout the can or mass of food.
If cans are heated from the outside, as would be the case if they are submerged within a retort, the larger the can, the longer it will take to heat the center portion of the can to any desired temperature. However, there are several other factors besides the size and shape of a can that affect heat penetration into the food within it. Principal among these factors is the nature and consistency of the food itself. This will determine, for example, whether heat will reach the center by straight conduction or will be speeded by some convection within a can.
Conduction and Convection Heating
Heat energy is transferred by conduction, convection, and radiation. In retorts used in canning, conduction and convection are important. Conduction is the method of heating in which the heat moves from one particle to another by contact, in more or less straight lines. In the case of conduction, the food does not move in the can and there is no circulation to stir hot food with cold food. Convection, on the other hand, involves movement in the mass being heated. In natural convection the heated portion of the food becomes lighter in density and rises; this sets up circulation within can. This circulation speeds temperature rises of the entire contents of the can. Forced convection occurs when circulation is prompted mechanically.
A liquid food such as canned tomato juice can be readily set into convection heating motion in addition to the heating by conduction it receives through the can wall. On the other hand, a solid food such as corned beef hash is too viscous to circulate, and so it will be virtually completely heated by conduction through the can wall and through itself. A product containing free liquid and solid, such as a can of pears within a sugar syrup, will be intermediate and will rise in temperature from a combination of conduction and convection; conduction through the fruit and convection from the moving syrup. Convection heating is far more rapid than conduction heating and so, other things being equal, if cans of these three products were placed in the same retort, uniform complete heating would be expected to be reached first in the tomato juice, second in the canned pears, and last in the corned beef hash.
Cold Point in Food Masses
When heat is applied from the outside, as in retorting, the food nearest the can surfaces will reach sterilization temperature sooner than the food nearer the center of the can. The point in a can or mass of food which is last to reach the final heating temperature is designated the “cold point” within the can or pass.
In a can of solid food heated by conduction the cold point is located in the very center of the can. However, in foods that undergo convection heating, unless the cans are agitated, the cold point is somewhat below the dead center of the can. To ensure that commercial sterilization is achieved, sufficient time must be allowed for the cold point of cans to reach the sterilization temperature and remain there for the required time interval to destroy the most resistant bacterial spores. If 12 D values are indicated and this corresponds, for example, to 121C for 2.5 min in a particular system, and if we ensure that the cold point of cans receives 121 C for 2.5 min, or an equivalent heat treatment, we are assured that every other region within the can has been adequately heated.
Determining Process Time and Process Lethality
The time required in a retort to produce lethal temperatures at the cold point can be determined with a heat-sensing thermocouple. Figure 8.5 indicates proper placement of the thermocouple to measure temperature at the cold points in canned foods that heat by conduction and natural convection. The cans with thermocouples are file with the particular food under study, sealed, and placed in the retort. After steam is admitted to the retort, the temperature rise is recorded with time. In a particular retort filled with a given number of cans of specified size and contents, it may require 30-40 min for the cold point of cans to reach a lethal temperature close to 121C, is due to the “comp-up” time required for the retort to reach processing temperature plus the time for heat penetration into the cans. The addition of the needed hold time completes the sterilization requirement.
Although a lethal effect at the cold point equivalent, for example, to 121 C for min may be called for, this degree of lethality can be achieved by various equivalent time-temperature exposures. Further, since the temperature rise during heat penetration of the can also accomplishes a degree of microbial destruction, this is common accounted for the decreasing the required holding time accordingly.
Figure: 8.5
Once treated sufficiently, cans are quickly cooled to prevent additional heat damage to the food. Because cooking is not instantaneous, some additional microbial destruction also occurs during the cooling period. Thus, to calculate effective retort process treatments, accurate heat penetration and cooking curves must be established. Total lethality of the process then represents a summation of the lethal effects of changing temperatures with time during the entire retort operation.
To perceive how total lethality of a process is calculated, one must first understand what is meant by the term “unit of lethality”. For heat process calculations, a unit of lethality has been defined as the heat kill equivalent to 1 min at 121 C against an organism of a given z value. All equally destructive heat treatments provide a unit of lethality. Further, fractions of a minute at 121C, or their equivalents, represent corresponding fractions of a unit of lethality. These fractions are referred to as “lethal rates.” We can calculate the lethal rate of any temperature reached at the cold point of a can being retorted, for any target organism, from the following relationship: lethal rate = antilog (T-250/z), where T is the temperature of the cold point in the container in degrees Fahrenheit and z for the target organism also is in degrees Fahrenheit. Similarly, lethal rate = antilog [(T-121/Z], where T and z are in degrees Celsius. These lethal rates, corresponding to successive temperatures taken from the heat penetration and cooking curves of a retort process are integrated to determine the total lethality of the process, which is its sterilization values F values. This may be done by plotting lethal rates against corresponding to one unit of lethality gives total lethality of F. In Figure 8.6 equals 9.74 min against an organism with a z value of 10 C. In Fig 8.6, lethal rates increase and then begin to decrease after about 30 min, which is the time when retort steam was turned off and cooling water turned on. The dotted line traced parallel to the descending line encloses an area corresponding to the retort process had the steam been turned off after 25.5 min (vertical dotted line). In this case, F the total lethality or sterilization value of the process, would equal 6.3.
Figure: 8.6
Since come-up time and penetration time will vary between different retorts, different size and shape of cans or bottles, and different compositions of foods, it is obvious that the required heat treatment will be different for each specific case. More advanced mathematical methods than those discussed here have been devised for the calculation of safe but not excessive process times and the effects of changes in processing on lethality. These calculations may be performed by computers which further control retort processes in highly instrumented canning plants. In all cases, however, bacterial death curve data, heat penetration properties of the food and certain characteristics of the retort must be known if an optimum process is to be calculated. Of course, a great deal of experience has been gained by the canning industry over the years and simple tables of heat treatments for well-known foods in common can sizes can be found in appropriate canning references (Table 8.2). However, when a new product is developed or when new packaging shapes or materials are employed, then specific determinations of effective heat treatments must be made.
PROTECTIVE EFFECTS OF FOOD CONSTITUENTS
Several-constituents of foods protect microorganisms to various degrees against heat. For example, sugar in high concentration protects bacterial spores, and canned fruit in a sugar syrup generally requires a higher temperature or longer time for sterilization than the same fruit without sugar. Starch and protein in foods generally act somewhat like sugar. Fats and oils have a great protective effect on microorganisms and their spores by interfering with the penetration of wet heat. As has been noted, wet heat at a given temperature is more lethal than dry heat, because moisture is an effective conductor of heat and penetrates into microbial cells and spores. If microorganisms are trapped within fat globules, then moisture can less readily penetrate into the cells and heating becomes more like dry heat. In the same can or food mass, organisms in the liquid phase may be quickly killed while more heating time is required for inactivation of the oil-phase flora.
This makes sterilization of meat products and fish packed in oil very difficult; the serve heat treatments required often adversely affect other food constituents. Likewise, because there is more fat and more sugar in ice cream mix than there is in milk, ice cream mix must be pasteurized at a higher temperature or for a longer time than milk to accomplish equivalent bacterial destruction.
In addition to any direct protective effects food constituents may on microorganisms, there are indirect effects related to differences in heat conductivity rates through different food materials. Fat, for example, is a poorer conductor of heat than is water. Further, and often more important, are the effects related to food consistency and its influences on whether conduction or convection heating will take place. If sufficient starch or other thickener is added to a food composition to convert it from a convection heating system to a conduction heating system, then in addition to any direct microbial protection, there will be a slowing down of the heat penetration rate to the old point within the container or food mass, and this will protect microorganisms. Because common starches in solution thicken upon heating, food supplemented with starch have a reduced rate of convection within cans during retorting and require longer retort times. Special starches have been developed which do not thicken on early heating but instead thicken on later heating or on cooling. Foods supplemented with these starches retain maximum convection rates in the retort, permitting shortened retort times and less heat damage. Then upon cooling, the starch imparts the desired thickening. In a typical application, a product like chow Mein can be heated with less softening of the vegetables from excessive heating yet possess the desired viscosity in the liquid phase.
The size and type of container that holds the food during thermal processing can also affect the sterilization process. Thin flexible pouches allow faster heat penetration into the cold center when compared to a cylindrical shape of a can, for example. This means that less heat is required for an equivalent lethality in pouches. Often higher quality product can be achieved from pouches than cans but at a higher container cost. The heat transfer properties of the container can also affect processing time. Thus, metal cans transfer heat more readily than plastic cans, resulting in shorter processing times.
INOCULATED PACK STUDIES
The many variables discussed so far make the determination of safe heat treatments by calculation alone difficult and sometimes risky, especially when applied to new products. In practice, therefore, formulas based on thermal death curves, heat penetration rates, and properties of specific retorts are used to gain an approximation of the safe heat treatment, but results are checked by what are termed inoculated pack studies.
In inoculated pack studies, a substantial population of a heat-resistant food spoilage organism such as PA 3679 is inoculated into cans of food, which are then processed in a retort. If formulas call for 60 min of heat, representative cans may be heated for 50,55,60,65, and 70 min. the cans are then stored at a temperature that would be favorable for growth of any surviving spores. The cans are periodically examined for, evidence of growth and spoilage, such as bulging from gas production (Fig. 8.7). Samples of no bulging can also be examined bacteriologically.
Figure: 8.7
The shortest heat treatment that consistently produces commercial sterility is then taken as the effective heat treatment to be used for subsequent commercial packs.
Different temperature-time combination
Different temperature-time combinations that are equally effective in microbial destruction can differ greatly in their damaging effect on foods. This is of the greatest practical importance in modern heat processing and is the basis for several of the more advanced heat preservation methods. If the time-temperature combinations required for destruction of C. botulinum in low-acid media are taken from thermal death curves, the following will be found be equally effective:
0.78 min at 127C 10 min at 116 C
1.45 min at 124 C 36 min at 110 C
2.78 min at 121 C 150 min at 104 C
5.27 min at 118 C 330 min at 100 C
This illustrates the simple relationship that the higher the temperature the less time is required for microbial destruction. This principle holds true for all types of microorganisms and spores. On the other hand, foods are not equally resistant to these combinations, and the more important factor in damaging the color, flavor, texture, and nutritional value of foods is long time rather than high temperature. If we were to inoculate milk with C. botulinum and then heat samples for 330 min at 100 C, 10 min at 116 C, and less than 1 min at 127 C, equal microbial destruction would occur in all three samples, but heat damage to the milk would be enormously different. The sample heated for 330 min would be thoroughly cooked in flavor and brown in color. The 10-min sample would be almost as bad. The 1-min sample, although still somewhat overheated, would not be far different from unheated milk. This difference in sensitivity to time and temperature between microorganisms and various foods is a general phenomenon. It applies to milk, meat, juices, and generally all other heat-sensitive food materials.
The greater relative sensitivity of microorganisms than food constituents to high temperature can be quantitatively defined in terms of different temperature coefficients for their destruction. Thus, whereas each increase of 10 C in temperature approximately doubles the rate of chemical reactions contributing to food deterioration, each 10 C increase, above the maximum temperature for growth, produces approximately a tenfold increase in the rate of microbial destruction. Since higher temperatures quality retention, high temperature short time heating treatments rather than low-temperature-long time heating treatments are used for heat-sensitive foods whenever possible.
In pasteurizing certain acid juices, for example, the industry formerly used treatments of about 63 C for 30 min. today, flash pasteurization at 88 C for 1 min, 100 C for 12 secs, or 121 C for 2 secs is the common practice. Although bacterial destruction is very nearly equivalent, the 121 C 2-sec treatment given the best quality juice with respect to flavor and vitamin retention. Such short holding times, however, require special equipment which is more difficult to design and generally is more expensive than that needed for processing at 63C.
HEATING BEFORE OR AFTER PACKAGING
The following principles very largely determine the design parameters for heat preservation equipment and commercial practices. The food processor will employ no less than that heat treatment which gives the necessary degree of microorganism destruction. This is further ensuring by periodic inspections from the FDA or equivalent local authorities. However, the food processor also will want to use the mildest effective heat treatment to ensure highest food quality, as well as to conserve energy.
It is convenient to separate heat preservation practices into two broad categories: one involves heating of food s in their final containers, the other employs heat prior to packaging. The latter category includes methods that are inherently less damaging to food quality, especially when the food can be readily subdivided (such as liquids) for rapid heat exchange. However, these methods then require packaging under aseptic or nearly aseptic conditions to prevent or at least minimize recontamination. On the other hand, heating within the package requires less technical sophistication and produces quite acceptable quality with the majority of foods; most canned foods are heated within the package.
Heating Food in Containers
Still Retort
One of the simplest applications of heating food in containers is sterilization of cans in a still retort, that is, the cans remain still while they are being heated. In this type of retort, temperature above 121 C generally may not be used or foods cook against the can walls. This is especially true of solid foods that do not circulate within the cans by convection, but it also can be a problem with liquid foods. Because 121 C is the upper temperature, and there is relatively little movement in the cans, the heating time to bring the cold point to sterilizing temperature is relatively long: for a small can of peas it may be 40 min.
Agitating Retorts
Processing time can be markedly reduced by shaking the cans during heating, especially with liquid or semiliquid foods. Not only is processing time shortened, but food quality is improved. This is accomplished with various kinds of agitating retorts, one type of which is shown in Fig. 8.8. Part of the wall has been cut away to show the cans resting in reels which rotate and thereby shake the contents. Forced convection within cans also depends on the degree of can filling, since some free headspace within cans is necessary for optimum food turnover within the cans. In addition to faster heating, there is less chance for food to cook onto the can walls since the can contents are in motion. Different types of agitation are possible; for example, cans may be made to turn end over end or to spin on their long axis. Depending on the physical properties of the food, one method may be more effective.
The reduction in processing times possible with agitating retorts compared with still retorts is seen in Table 8.3. These substantial reductions in time with associated quality advantages would not be realized in foods that heat primarily by conduction; for such foods the simpler and generally less costly still retorts may be quite satisfactory.
Pressure Considerations
Whether still or agitating retorts are used, the high temperatures required for commercial sterilization commonly are obtained from steam under pressure.
Steam pressure of approximately 10, 15, and 20 psi (above atmospheric pressure) are required for heating at 116 C, 121 C, and 127 C (1 psi = 0.07kg/cm2 = 6895 Pascal).
Moist foods in cans have part of their moisture converted to steam at these temperatures and produce equivalent pressures within the cans although there is little pressure difference between the inside and outside of the can when temperatures are equal. A special case is foods canned under vacuum. Then the initial pressure within a can will be less than the pressure in the retort to an extent determined by the degree of vacuum used at time of can closure. Control of pressure differences inside and outside of cans and other containers during and following heat treatment are of obvious importance to prevent mechanical damage to containers. Several techniques are employed to prevent such damage.
If the vacuum within cans is such that retort pressures cause can collapse, heavier gauge of steel may be required. More commonly pressure problems are due to greater pressures within the container than on its outside. This occurs when steam pressure is too rapidly released in closing down a batch-type retort to atmospheric pressure. The problem is greater in the case of glass jars than with cans; excessive internal pressure can easily blow the lids from glass jars since these generally have a weaker seal than the lids of cans. During retorting of glass jars, provisions are made for air pressure over a layer of water to balance internal and external pressures. Partial cooking of containers before releasing them from retorts is the common way to decrease internal container pressure. Many continuous retorts, such as the agitating type in Fig. 8.8, provide semi pressurized cooking zones following the heating zone just prior to release of cans to atmospheric pressure.
With the increasing use of flexible packaging materials has come the sterilization of foods in flexible plastic pouches. Here, pressure problems can be still greater than with glass jars. Overriding air pressure must be applied when the pouches are cooled after retorting so that the steam pressure inside the pouch does not cause the pouch to burst. In addition, a uniform heat treatment requires that the pouches be evenly exposed to the heating medium rather than be allowed to contact each other and pile up. One means of better controlling pouches during retorting is to sandwich the pouches between rigid supports (Fig 8.9). Plastic pouches require shorter retort times since heat penetration through the thin pouches is quite rapid. This, in turn, and produces high quality products and save on energy costs.
All plastic rigid “cans” are also being used to retort foods. These cans have the advantage that they can be reheated in a microwave oven. They require many of the same types of handling requirements as flexible pouches.
Hydrostatics Cooker and Cooler
Continuous retorts (usually of the agitating type) are pressure-tight and built with special valves and locks for admitting and removing cans from the sterilizing chamber. Without those, pressure conditions would not be held constant and sterilizing temperatures could not be closely controlled. Another type of continuous pressure retort, which is open to the atmosphere at the inlet and outlet ends, is the hydrostatic pressure cooker and cooler.
This type of heating equipment consists essentially of a “U” tube with an enlarged lower section. Steam is admitted to the enlarged section and hot water fills one of the leg of the “U” while cool water fills be the other leg (Fig. 8.10). Cans are carried by a chain conveyor done the hot water leg, through the steam zone, which may involve an undulating path to increase residence time, and up the cool water leg. These legs are sufficiently high to produce a hydrostatic head pressure to balance the steam pressure in the sterilizing zone. If a temperature of 127 C is used in the sterilizing zone, then this would be equal to a pressure of about 140,000 Pascal (20 psi) above atmospheric pressure which would be balanced by water heights of about 14 m (46 ft.) in the hot and cold legs.
Figure: 8.9 and Figure 8.10
As cans descend the hot water leg and enter the steam zone, their internal pressure increases as food moisture begins to boil. But this is balanced by the increasing external hydrostatic pressure. Similarly, as high-pressure cans pass through the water seal and ascend the cool water leg, their gradually reduced internal pressure is balanced by the decreasing hydrostatic head in this cool leg. In this way cans are not subjected to sudden changes in pressure. For this reason, the system also is well suited to the retorting of foods and beverages in jars and bottles.
Direct Flame sterilization
Where sterilizing temperatures above 100 C are needed, steam under pressure generally is the heat exchange medium, and vessels capable of withstanding pressure add to the cost of equipment. Another method, introduced from France, employs direct flame to contact cans as the cans are rotated in the course of being conveyed past gas jets. Excellent rates of heating are achieved with high product quality and reduced costs, but commercial experience with this type of system is still somewhat limited.
In-package Pasteurization
In-package heating need not be to the pint of sterilization or commercial sterilization. Tunnels of various designs are used to pasteurize food and beverages in cans, bottles, and jars. Hot water sprays or steam jets are directed at the containers and varying temperature zones progressing to cooking temperatures are commonly employed. Temperature changes must be gradual to prevent thermal shock to glass. Such systems are operated at atmospheric pressure. This is one of the methods of pasteurizing beer containers.
Heating Food Prior to Packaging
As already stated, there are advantages to heating heat-sensitive foods prior to packaging. These are related to the ability to heat rapidly by exposing food in a subdivided state to a heat exchange surface or medium, rather than having to allow appreciable time for heat penetration into a relatively large volume of food in a container.
Batch Pasteurization
One of the earliest and simplest methods, of effectively pasteurizing liquid foods, such as milk, is to heat the food in a vat with mild agitation. Raw milk commonly is pumped into a steam-heated jacketed vat, brought to temperature, held for the prescribed time, and then pumped over a plate-type cooler prior to bottling or cartooning. Milk must be quickly brought to 62.8 C (145 F), held at this temperature for 30 min, and rapidly cooled. In addition to destroying common pathogens, this heat treatment also inactivates the enzyme lipase, which otherwise would quickly cause the milk to become rancid. Batch pasteurization, also known as the holding method of pasteurization, is still widely practiced in some parts of the world, but it has largely given way to high-temperature-short-time continuous pasteurization.
High-Temperature-Short-Time Pasteurization
High-temperature-short-time (HTST) pasteurization of raw milk employs a temperature of at least 71.7 C (161 F) for at least 15 sec. This is equivalent in bacterial destruction to the batch method. In HTST
Figure;8.11
pasteurization (Fig. 8.11), raw milk held in a cool storage tank is pumped through a plate-type heat exchanger and brought to temperature. The key to the process rests in ensuring that every particle of the milk remains at not lower than 71.7 C for no less than 15 sec. this is accomplished by pumping the heated milk through a holding tube of such length and diameter that it takes every milk particle at least 15 secs to pass through the tube. At the end of the tube is an accurate temperature sensing device and valve. Should any milk reach the end of the holding tube and be down in temperature even one degree, a flow diversion valve checks this flow of milk and sends it back through the heat exchanger once again to be reheated. In this way no ilk escapes the required heat treatment. Frequent checks of the equipment are made by authorized milk inspectors to help ensure its proper operation. After emerging from the holding tube, the milk is cooled and may be cartooned or bottled. Cooking not only prevents further heat damage to the milk but also retards subsequent bacterial multiplication since the milk is not sterile.
Pasteurization by the HTST method is not limited to milk and is widely used in the food industry. However, times and temperatures vary in accordance with the effects of different foods on microorganism survival and the heat sensitivities of these foods.
Aseptic packaging
Aseptic packaging is a method in which food is sterilized or commercially sterilized outside of the can, usually in a continuous process, and then aseptically placed in previously sterilized containers which are subsequently sealed in an aseptic environment.
The most commercially successful form of aseptic packaging utilizes paper and plastic materials which are sterilized, formed, filled, and sealed in continuous operation. The package may be sterilized with heat or a combination of heat and chemicals. In some cases, the disinfectant property of hydrogen peroxide (H2O2) is combined with heated air or with ultraviolet light to make lower temperatures effective in sterilizing these less heat-resistant packaging materials. Coffee cream and coffee whiteners, for example, are packaged in small single-service paper packets this way as are larger volume size milk and juice products.
Quick heating of liquid foods may be done in a plat-type heat exchanger (see Fig. 5.7) or in a tubular scraped-surface heat exchanger (Fig. 8.12). This latter type consists essentially of a tube within a tub. Steam flows through the space between the tubes while food flows through the inner tube. The inner tube also is provided with a rotating shaft or motor equipped with scraper blades to prevent food from burning onto the heat exchange surface. In contact with the hot surface, the thin layer of food may be brought to sterilization temperature in 1 sec or less. Food temperatures employed may be as high as 150 C and sterilization takes place in 1 or 2 sec, yielding food products of the highest quality, and often with significant energy saving. If it is desired to prolong residence time beyond this, then a holding tube is added as in the case of HTST pasteurization. Such rapid sterilization at extremely high temperature is referred to as ultrahigh-temperature (UHT) sterilization.
The sterile food must be quickly cooled to room temperature, because at these high temperatures product quality can be impaired in seconds. Quick cooling can be accomplished with the same types of plate or tubular scraped-surface heat exchangers, used with refrigerants instead of steam.
Figure: 8.12
Aseptic packaging is also used with metal cans as well as large plastic and metal drums or large flexible pouches (Fig. 8.13). Great quantities of food materials are used as intermediates in the production of further processed foods. This frequently requires packaging of such items as tomato paste or apricot puree in large containers such as 55-gal drums, as smaller units involve greater expense. The food manufacturer then may use the tomato paste in the production of ketchup or the apricot puree in bakery products. If such large volumes were to be sterilized in drums, by the time the cold point reached sterilization temperature the product nearer the drum walls would be extensively burned. Such items can be quickly sterilized in efficient heat exchangers and aseptically packaged. In this case, large chambers have been developed in which the drums and lids are sterilized under superheated steam and then filled with the product and sealed aseptically within the chamber. This technology has advanced to the point where sterile food can be aseptically filled into previously sterilized silo tanks and tank cars. More will be said about aseptic packaging in Chapter 21.
Hot Pack or Hot Fill
The terms hot pack or hot fill refer to the packing of previously pasteurized or sterilized foods, while still hot, into clean but not necessarily sterile containers, under clean but not necessarily aseptic conditions. The heat of the food and some holding period before cooking the closer container is utilized to render the container commercially sterile.
Hot pack, as distinguished from aseptic packaging, is most effective with acid foods since lower temperatures in the presence of acid are lethal, further, at a ph of 4.6 Clostridium botulinum will not grow or produce toxin, so this health hazard is not present. Hot pack with low-acid foods (above ph 4.6) is not feasible unless the product is recognized as being only pasteurized and will be stored under refrigeration or unless the hot pack treatment is combined with some additional means of preservation such as a very high sugar content.
Figure: 8.13
This is because the residual heat of the food in the absence of appreciable acid is not sufficient to guarantee destruction of spores that may be present on container surfaces or that may enter containers during filling and sealing. Even with acid foods, very definite food temperatures and holding times in the sealed containers before they are cooled for warehouse storage must be adhered to for hot pack processing to be effective. These temperatures and times depend on the specific product’s ph and other food characteristics.
In home canning, when fruit and sugar are boiled together to make jam and the hot jam is poured into jars that have been previously boiled, the principle of hot pack is being employed. Home canning instructions further call for inverting the filled jars after a short time. This is to ensure that the hot acid product contacts all surfaces of the jar lid for sterilization. However, for home canning of meats and other low-acid foods, direction always call for pressure cooking of closed containers as is done in conventional commercial retorting.
In commercial practice, acid juices such as orange, grapefruit, grape, tomato, and various acid fruits and vegetables, such as sauerkraut, commonly are hot packed following prior pasteurization or sterilization. Typically, acid fruits and juices are first heated in the range of about 77-100 C for about 30-60 min, hot filled at no lower than 77 C and often closer to 93 C, and held at this temperature for 1-3 min, including an inversion before cooking. In the case of tomato juice a common practice is HTST heating of juice at 121 C for 0.7 min, cooking below the boiling point but hot below 91 C for hot fill can sterilization, and can holding for 3 min, including an inversion before final cooking. Precise times and temperatures depend on the ph of the particular tomato juice batch and can be confirmed by inoculate pack studies.
Microwave Heating
Microwave energy produces heat in materials that absorb it. Microwave energy and energies of closely related frequencies are finding ever-increasing applications in the food industry. These include heat preservation. Microwave energy heats foods in a unique fashion that largely eliminates temperature gradients between the surface and center of food masses. Foods do not heat from the outside to the inside as with conventional heating since microwave penetration can generate heat throughout the food mass simultaneously. In this case the concept of clod point and the limitations of conventional heat penetration rates are not directly applicable. The use of microwaves can result in very rapid heating but requires special equipment, and often specific packaging materials, since microwaves will not pass through metal cans or metal foils. Microwave heating also can produce major differences in food appearance and other properties compared with the more conventional methods of heating. More will be said about microwave heating in Chapter 11.
GOVERNEMTN REGULATION
In the United States, as elsewhere, the Food and Drug Administration requires thermal processing to be carried out under what is known as Good Manufacturing Practices (GMPs) to help assure food safety and wholesomeness. Among these GMPs are specific regulations pertaining to low-acid canned foods (foods that are thermally processed, have ph values greater than 4.6 and water activity greater than 0.85, are packaged in hermetically sealed container, and are not stored under refrigeration).
FDA has issued a number of regulations for low-acid foods. Additional regulations for acidified foods have also been released. The primary purpose of these regulations is to describe safe procedures for manufacturing, processing, and packing of foods that could otherwise support the growth of and toxin production by Clostridium botulinum. The safety of low-acid and acidified foods is further ensured through the Emergency Permit Control regulations, which require manufacturers to register their processing plants and file their processes with the FDA. These regulations also require firms to adhere to their filed and approved processes, to maintain detailed records, and to make these records available to authorized FDA personnel. Since differences in processing equipment, operating conditions, container type or size, kind of food, and food form constitute different processes, presently over 100,000 processes have been filled with the FDA under these regulations.
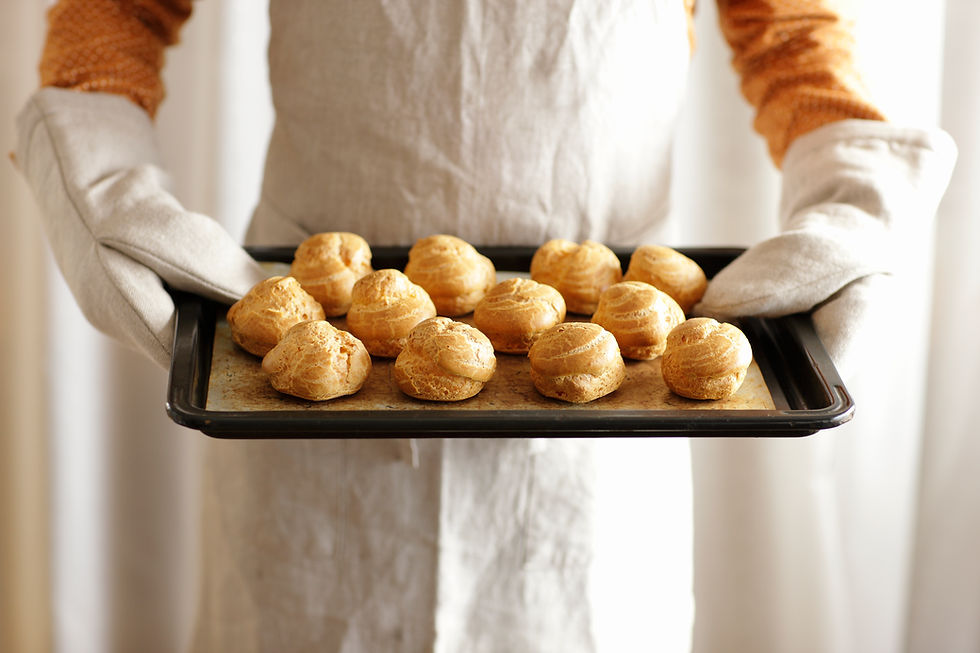
Comments