Vegetables and fruits have many similarities with respect to their compositions, methods of cultivation and harvesting, storage properties, and processing. In fact, many vegetables are considered fruits in the true botanical sense. Botanically, fruits are those portions of a plant that house seeds. Therefore, tomatoes, cucumbers, eggplant, peppers, okra, sweet corn, and other vegetables would be classified as fruits according to this definition. However, the important distinction between fruits and vegetables has come to be made on a usage basis: those plant items that are generally eaten with the main course of a meal are often considered to be vegetables; those that commonly are eaten alone or as a dessert are considered fruits. This is the distinction made by food processors, certain marketing laws, and the consuming public.

GENERAL PROPERTIES
Because vegetables are derived from various parts of plants, it is sometimes helpful to classify vegetables according to the plant part from which they are derived, such as roots, leaves, stems, buds, and so on (Table 18.1)
Fruits are the mature ovaries of plants with their seeds. The edible portion of most fruits is the fleshy part of the pericarp or vessel surrounding the seeds. Fruits, in general, are acidic and sugary. They commonly are grouped into several major divisions, depending principally on botanical structure, chemical composition, and climatic requirements. Thus, berries are generally small and quite fragile, although cranberries are rather tough. Grapes are also berries, which grow in clusters. Melons, on the other hand, are large and are known as “drupes.” “Pomes” contain many pits and are represented by apples, quince, and pears. Citrus fruits, characteristically high in citric acid, include oranges, grapefruit, and lemons. Tropical and subtropical fruits include bananas, dates, figs, pineapples, papayas, mangos, and others, but not the separate group of citrus fruits; these all require warm climates for growth.
GROSS CONSUMPTION
The compositions of representative vegetables and fruits in comparison with a few of the cereal grains are shown in Table 18.2. the composition of vegetables and fruits depends not only on botanical variety, cultivation practices, and weather but also on the degree of maturity prior to harvest and the conditions of ripeness, which continues after harvest and is influenced by storage conditions. Nevertheless, some generalizations can be made.
Most fresh vegetables and fruits are high in water, low in fat. The water content is generally greater than 70% and frequently greater than 85%. Interestingly, the water content of milk and apples is similar. Commonly, protein content is no greater than 3.5% and fat content no greater than 0.5%. exceptions exist to these typical values: dates and raisins are substantially lower in moisture but cannot be considered fresh in the above sense; legumes such as peas and certain beans are higher in protein; a few vegetables such as sweet corn are slightly higher in fat; and avocados are substantially higher in fat. On the other hand, vegetables and fruits are important sources of both digestible and ingestible carbohydrates. The digestible carbohydrates are present largely as sugars and starches, and the indigestible cellulosic and peptic materials provide fiber, which is important to normal digestion. Fruits and vegetables are important sources of mineral and certain vitamins also, especially vitamins A and C. the precursors of vitamin A, including beta-carotene and certain other carotenoids, are present particularly in the yellow-orange fruits and vegetables and in the green, leafy vegetables. Citrus fruits and excellent sources of vitamin C, but green, leafy vegetables, and tomatoes are also good sources. Potatoes also are an important source of vitamin C in many countries, not so much because of the level of vitamin C in potatoes, which is not especially high, but rather because of the large quantities of potatoes consumed.
STRUCTURAL FEATURES
The structural unit of the edible portion of most fruits and vegetables is the parenchyma cell (Fig.18.11).
Figure 18.1
Although parenchyma cells of different fruits and vegetables differ somewhat in gross size and appearance, all have essentially the same fundamental structure. Parenchyma cells of plants differ from animal cells in that the actively metabolizing protoplast portion of plant cells represents only a small fraction (about 5%) of the total cell volume. This protoplast is rather film like and is pressed against the cell wall by the large water-filled central vacuole. The protoplast has inner and outer semi-permeable membrane layers between which are confined the cytoplasm and its nucleus. The cytoplasm contains various inclusions, among them starch granules and plastids such as the chloroplasts and other pigment-containing chloroplasts. The cell wall, cellulosic in nature, contributes rigidity to the parenchyma cell and confines the outer protoplasmic membrane. It also is the structure against which other parenchyma cells are cemented to form extensive three-dimensional tissue masses. The layer between cell walls of adjacent parenchyma cells, referred to as the middle lamella, is composed largely of pectin and polysaccharide materials which act to cement cells together. Air spaces also exist, especially at the angles formed where several cells come together.
the relationships between these structures and their chemical compositions are further indicated in Table 18.3. Parenchyma cells vary in size from plant to plant but are quite large when compared to bacterial or yeast cells. The larger parenchyma cells may have volumes many thousand times greater than a typical bacterial cell.
Several types of cells other than parenchyma cells contribute to the familiar structures of fruits and vegetables. These include various types of tube like conducting cells, which distribute water and salts throughout the plant. Such cells produce fibrous structures toughened by the presence of cellulose and the wood like substance lignin. Cellulose, lignin, and pectin substances also occur in specialized supporting cells, which increase in importance as plants become older. An important structural feature of all plants, including fruits and vegetables, is protective tissue. This can take many forms but usually is made up of specialized parenchyma cells that are pressed compactly together to form a skin, peel, or rind. Surface cells of these protective structures on leaves, stems, or fruits secrete waxy cut in and form a water-impermeable cuticle. These surface tissues, especially on leaves and young stems, also contain numerous valve like cellular structure (stomata) through which moisture and gasses can pass.
Turgor and Texture
The range of textures encouraged in fresh and cooked vegetables and fruits is great and, to a large extent, can be explained by changes in specific cellular components. Since plant tissues generally contain more than two-third water, the relationships between these components and water further determine textural difference.
Cell Turgor
Turgor is the rigidity of plant cells resulting from being filled with water. The state of turgor, which depends on osmotic forces, is the most important factor determining the texture of fruits and vegetables. The cell walls of plant tissues have varying degrees of elasticity and are largely permeable to water and ions as well as to small molecules. The membranes of the living protoplast are semi-permeable; that is, they allow passage of water but selectively transfer dissolved and suspended materials. The cell vacuoles contain most of the water of plant cells; within this water are dissolved sugars, acids, salts, amino acids, some water-soluble pigments and vitamins, and other low-molecular-weight constituents.
In the living plant, water taken up by the roots passes through the cell walls and membranes into the cytoplasm of the protoplasts and into the vacuoles to establish a state of osmotic equilibrium within the cells. the osmotic pressure within the cell vacuoles’ and within the protoplasts pushes the protoplasts against the cell walls and causes them to stretch slightly in accordance with their elastic properties. These processes result in the characteristic appearance of live plants and are responsible for the desired for the desired plumpness, succulence, and much of the crispness of harvested live fruits and vegetables.
When plant tissues are damaged or killed by storage, freezing, cooking, or other causes, denaturation of the proteins of the cell membranes occurs, resulting in the loss of perm selectivity. Without perm-selectivity, osmotic pressure in cell vacuoles and protoplasts cannot be maintained, and water and dissolved substances are free to diffuse of the cells and leave the remaining tissue in a soft and wilted condition.
Other Factors Affecting Texture
Whether a high degree turgor exists in live fruits and vegetables or a relative state of softness develops from loss of osmotic pressure, final texture is further influenced by several cell constituents.
Cellulose, Hemicellulose, and Lignin. Cell walls in young plants are very thin and are composed largely of cellulose. As the plant ages, cell walls tend to thicken and become higher in hemicellulose and in lignin. these materials are fibrous and tough and are not significantly softened by cooking.
Pectic Substances. Pectic and related substance are complex polymers of sugar acid derivatives. The cement like substance found especially in the middle lamella, which helps hold plant cells to one another is a water insoluble pectic, which can form gels or viscous colloidal suspensions with sugar and acid. Certain water-soluble pectic substances also react with metal ions, particularly calcium, to form water-insoluble salts such as calcium pectates. The various pectic substances may influence texture of vegetables and fruits in several ways. When vegetables or fruits are cooked, some of the water-insoluble pectic substances in hydrolyzed into water-soluble pectin. This results in a degree of cell separation in the tissues and contributes to tenderness. Since many fruits and vegetables are somewhat acidic and contain sugars, the soluble pectin also tends to form colloidal suspensions which thicken the juice or pulp of these products.
Fruits and vegetables also contain a natural enzyme that can further hydrolyze pectin to the extent that it loses much of its gel-forming property. This enzyme is known as pectin methyl esterase. Some products (e.g., tomato juice and tomato paste) contain both pectin and pectin methyl esterase. If freshly prepared tomato juice or paste is allowed to stand, the original viscosity gradually decreases due to the action of pectin methyl esterase on pectin gel. This can be prevented if the tomato products are quickly heated to a temperature of about 82C to inactivate enzyme liberated from broken cells before the pectin is hydrolyzed. This treatment, known as the hot-break process, is commonly practiced in the manufacture of tomato paste and tomato juice products to yield products of high viscosity. In contrast, when low-viscosity products are desired, no heat is used and enzyme activity is allowed to proceed. This is the cold break process. After the appropriate viscosity is achieved, the product can be heat treated, as in canning, to preserve it for long-term storage.
It often is desirable to firm the texture of fruits or vegetables, especially when products are normally softened by processing. In this case, advantage is taken of the reaction between soluble pectic substances and calcium ions to form calcium pectates. These calcium pectates are water insoluble; when they are produced within the tissues of fruits and vegetables, they increase structural rigidity. Thus, it is common commercial practice to add low levels of calcium salts to tomatoes, apples, and other vegetables and fruits prior to canning or freezing.
Starch. The occurrence of starch within starch granules and the swelling and gelatinization of these granules in the presence of moisture and heat have previously been mentioned. When starch granules absorb water and gelatinize, they gradually lose their granular structure and produce a pasty, viscous colloidal suspension. The swelling of starch granules within the cells of plant tissues on heating causes a corresponding swelling of these cells and contributes to firm texture and plumpness.
On the other hand, starch swelling together with osmotic pressure can be so great as to cause plant cells to burst. When this happens, the viscous colloidal starch suspensions oozes from the cells and imparts pastiness to the system. The same occurs when cells containing much starch are ruptured by processing condition. This is particularly important in the case of potato products. The desirable texture of mashed potatoes and other potato products in a mealiness rather than a stickiness or pastimes. Therefore, in the production of dehydrated potato granules and flakes much of the technology of mixing and drying is aimed at minimizing both cell rupture and release of free starch. The same is true in the cooking and mashing of fresh potatoes, which, if excessive, can produce undesirable pastiness.
Color and Color Changes
Much of the appeal of fruits and vegetables in our diets is due to their desirable colors. The pigments and color precursors found in fruits and vegetables occur for the most part in the cellular plastid inclusions (e.g., chloroplasts and other chromo plast) and to a lesser extent dissolved in fat droplets or water within the cell protoplast and vacuole. These pigments are classified into four major groups chlorophylls, carotenoids, anthocyanin, and anthoxanthins. Pigments belonging to the latter two groups also are referred to as flavonoids, and include the tannins.
Chlorophylls
Chlorophylls are largely contained within the chloroplasts and have a primary role in the photosynthetic production of carbohydrates from carbon dioxide and water. The bright green color of leaves and other plant parts is due largely to oil-soluble chlorophylls, which in nature are bound to protein molecules in highly organized complexes. When plant cells are killed by ageing, processing, or cooking, the proteins are denatured and the magnesium bound in the chlorophyll may be released. This causes a chemical change of chlorophyll to pheophytin which is olive green or brown in color. Conversion to pheophytin is favored by acid pH and occurs less readily under alkaline conditions. For this reason, peas, beans, spinach, and other green vegetables, which tend to lose their bright green colors on heating, can be to some extent protected against such color changes by the addition of sodium bicarbonate or other alkali to the cooking or canning water. The addition of magnesium salts can help reduce the conversion of chlorophyll to pheophytin. However, this practice is not looked on favorably nor used commercially because alkaline pH tends to soften cellulose and vegetable texture and to increase the destruction of vitamin C and thiamin at cooking temperatures.
Carotenoids
Pigments belonging to the carotenoid group are fat soluble and range in color from yellow through orange to red. They often occur along with the chlorophylls in the chloroplasts but are present in other chromo plast also and may occur free in fat droplets. Important carotenoids include the orange carotenes of carrot, corn, apricot, peach, citrus fruits, and squash; the red lycopene of tomato, watermelon, and apricot; the yellow-orange xanthophyll of corn, peach, paprika, and squash; and the yellow-orange Croce tin of the spice saffron. These and other carotenoids seldom occur singly within plant cells.
Of major importance is the relationship of some carotenoids to vitamin A. some carotenoids serve as precursors to vitamin A. A molecule of orange beta-carotene is converted into two molecules of colorless vitamin A within the body. Some other carotenoids (e.g., alpha-carotene, gamma-carotene, and kryptoxanthin) are precursors of vitamin A also, but because of minor differences in chemical structure one molecule of each of these yields only one molecule of vitamin A.
In food processing, the carotenoids are fairly resistant to heat, changes in pH, and water leaching since they are fat soluble. However, they are very sensitive to oxidation, which results in both color loss and destruction of vitamin A activity.
Anthocyanin
Anthocyanin pigments belong to a group of plant chemicals known as flavonoids. They are water soluble and commonly are present in the juices of fruits and vegetables. The anthocyanin includes the purple, blue, and red pigments of grapes, berries, plums, eggplant, and cherries. The color of anthocyanin depends on the pH thus, many of the anthocyanin that are violet or blue in alkaline media become red on addition of acid. The color of red fruits and vegetables shifts toward violet and gray-blue if the pH becomes basic. Red anthocyanin also tends to become more violet, blue, or colorless on reaction with metal ions, which is one reason for lacquering the inside of metal cans when the tur color of anthocyanin-containing fruits and vegetables is to be preserved. The water solubility of anthocyanin also results in easy leaching of these pigments from cut fruits and vegetables during processing and cooking.
Flavonoids
The yellow flavonoids are structurally related to anthocyanin and comprise a large group of chemicals which are widely found in plant foods. they also are pH sensitive, tending toward a deeper yellow in alkaline media. Thus, potatoes or apples become somewhat yellow when cooked in water with a pH of 8 or higher, which is common in many areas. Acidification of the water to pH 6 or lower favors a whiter color.
Tannins
Tannins are complex mixtures of phenolic compounds found in plants. Under most circumstances they are colorless, but on reaction with metal ions they form a range of dark-colored complexes which may be red, brown, green, gray, or black. They are responsible for the dark color found in the bark of oak, sumac, and myrobalen trees. The various shades of these colored complex, and other factors not yet fully understood.
Water-soluble tannins appear in the juices squeezed from grapes, apples, and other fruits as well as in the brews extracted from tea and coffee. The color and clarity of tea are influenced by the hardness and pH of the brewing water. Alkaline waters that contain calcium and magnesium favor the formation of dark brown tannin complexes, which precipitate when the tea is cooled. If acid in the form of lemon juice is added to such tea, its color lightens and the precipitate tends to dissolve. Iron from equipment or from pitted cans has caused a number of unexpected colors to develop in tannin containing products, such as coffee, cocoa, and foods flavored with these.
The tannins also are important because they possess astringency which influences flavor and contributes body to coffee, tea, wine, apple cider, beer, and other beverages. Excessive astringency causes a pucker sensation in the mouth, which is the condition produced when tea becomes high in tannins from over brewing.
Betalains
Like anthocyanin, Betalains are red water-soluble pigments but are chemically different and are less widely dispersed in the plant world. The primary food plant in which they occur is the red beet. They also occur in some cactus fruits and flowers. Betalains are degraded by thermal processing but occur in such high amounts that sufficient pigments remain for coloration. They are relatively stable compared to other natural red pigments, especially in the pH range of 4-6. They have been considered for use as naturally occurring food colorants.
ACTIVITIES OF LIVING SYSTEMS
Fruits and vegetables continue to respire after harvest and, thus, can be considered “alive.” Respiration means the produce takes in oxygen and given off carbon dioxide, moisture, and heat, which influence storage, packaging, and refrigeration requirements. Moisture and heat can build up in storage and packaging causing the growth of molds. Enough heat can be generated to damage the produce itself.
Fruits and vegetables, before and after harvest, also undergo changes in carbohydrates, pectin, and organic acids, which influence the various quality attributes of the products. Few generalizations can be given concerning changes in starches and sugars. In some plant products, sugars decrease quickly and starch increases soon after harvest. This is the case for ripe sweet corn, which can suffer flavor and texture quality losses in a very few hours after harvest. Unripe fruit, in contrast, frequently is high in starch and low in sugars. Continued ripening after harvest generally results in a decrease in starch and an increase in sugars and occurs in apples and pears. However, this does not necessarily mean that the starch is the source of the newly formed sugars. Further, changes in starch and sugars are markedly influenced by postharvest storage temperatures. For example, potatoes stored below about 10C continue to build up high levels of sugars, whereas the same potatoes stored above 10C do not. Thus, potatoes that are stored for dehydration are kept above 10C in order to keep the level of reducing sugars low and to minimize Maillard browning reactions during drying and subsequent storage of the dried product.
After harvest, the changes in pectin of fruits and vegetables are more predictable. Generally, these is a decrease in water-soluble pectin substance and a corresponding increase in water-soluble pectin. This contributes to the gradual softening of fruits and vegetables during storage and ripening. Further breakdown of water-soluble pectin by pectin methyl esterase also occurs.
The organic acids of fruits generally decrease during storage and ripening. This occurs in apples and pears and is especially important in oranges. Oranges have a long ripening period on the tree, and time of pickling is largely determined by degree of acidity and sugar content, which have major effects on juice quality.
As acids disappear during ripening, more than just tartness of fruits is affected. Since many plant pigments are sensitive to acid, fruit color would be expected to change as the organic acid content changes. Additionally, the viscosity of pectin gel is affected by acid and sugar, both of which change in concentration with ripening. Further consequences of the live state of vegetables and fruits are indicted in the following sections on harvesting and processing.
The quality decline in stored respiring fruits and vegetables is termed senescence and results from the continued enzymatic activity. A number of factors influence respiration rate and, hence, senescence rate. The two primary factors for a given produce item are temperature and the composition of the storage atmosphere. Reduced temperatures, lower oxygen levels, and raised carbon dioxide levels can be combined to greatly reduce the rate of senescence and increase storage time. This is not without limit. Many fruits and vegetables suffer “chill injury” from temperatures that are too low. If the carbon dioxide levels raise above 5-10%, damage can also occur. Storage of produce under such controlled conditions is termed controlled atmosphere storage and commonly practiced for some produce such as apples.
HARVESTING AND PROCESSING OF VEGETABLES
Varietal Differences
The food scientist and vegetable processor must appreciate the substantial differences that cultivar of a given vegetable possess. In addition to differences in response to weather and in pest resistance, cultivars of a given vegetable differ in size, shape, time of maturity, and resistance to physical damage. These latter factors are of the greatest importance in the design and use of mechanical harvesting devices. Varietal difference is also important in the processing of fruits and vegetables. Certain types of apples become soft and loose texture more readily than others when heated. These apples might be more desirable for applesauce but less desirable for products such as pie fillings. Varietal differences in the effects of processing are important for most fruits and vegetables.
A varietal difference in resistance to tomato cracking is illustrated in Fig. 18.2. varietal differences in the effects of processing are important for most fruits and vegetables.
A vertical difference in resistance to tomato cracking is illustrated in Fig. 18.2 vertical difference in warehouse storage stability and in suitability for different processing methods also exist. A cultivar of peas that is suitable for canning may be quite unsatisfactory for freezing, and cultivars of potatoes that are preferred for freezing may be less satisfactory for drying or potato chip manufacture. This should be expected since different cultivars of a given vegetable vary somewhat in chemical composition, cellular structure, and biological activity of their enzyme systems. Because of the importance of varietal differences, large food companies commonly provide special seed to farmers whose crops they contract to buy a year in advance. They also frequently manage their own vegetable farms to further guarantee a sufficient supply of high quality uniform raw materials.
Figure 18.2
Harvesting and Preprocessing Considerations
When vegetables are maturing in the field, they are changing from day to day. There is a time when the vegetable will be at peak quality from the standpoint of color, texture, and flavor. Because this peak quality lasts only briefly, harvesting and processing of several vegetables, including tomatoes, corn, and peas, are rigidly scheduled to capture this peak quality.
After a vegetable is harvested, it may quickly pass beyond the peak quality condition. This is independent of microbial spoilage. One study on sweet corn showed that in just 24h at room temperature 26% of the total sugars were lost with a comparable loss of sweetness in the corn. Even when stored just above freezing at 0C, 8% of the sugar was lost in 24 h and 22% in 4 days. Some of this sugar was probably converted to starch; some was used during respiration. In similar fashion, peas and lima beans can lose over 50% of their sugar in just 1 day at room temperature; losses are slower under refrigeration but there is still a great change in vegetable sweetness and freshness of flavor within 2 or 3 days. Not all losses of sugar are due to respiration or conversion to starch. Some of the sugar in asparagus can be converted to fibrous tissue after harvest; this contributes to a woodier texture.
Along with the loss of sugar, the evolution of heat can be a serious problem when large stockpiles of vegetables are transported or held prior to processing. At room temperature, some vegetables will liberate heat at a rate of 60 Btu/lb./day. This is enough for each ton of vegetables and speeds growth of microorganisms; the harvested vegetables must be cooled if not processed immediately.
But cooling only slows down the rate of deterioration, it does not prevent it, and vegetables differ in their resistance to cold storage. As pointed out earlier, each type of vegetable has its optimum cold storage temperature which may be between about 0 and 10C. storage below 7C in the case of cucumbers, for example, will result in pitting, soft spots, and decay. What actually happens is that at too low a temperature the normal metabolism of the living vegetable is altered and various abnormalities occur along with decreased resistance to invasion by microorganisms that are present and can grow at the low storage temperature.
The continual loss of water by harvested vegetables due to transpiration, respiration, and physical drying of cut surfaces results in wilting of leafy vegetables, loss of plumpness of fleshy vegetables, and loss of weight of both. Moisture loss cannot be completely and effectively prevented y hermetic packaging. When fresh vegetables and sealed in plastic bags, the bags become fogged with moisture, the carbon dioxide level increases, and oxygen decreases. Because these conditions accelerate the deterioration of certain vegetables, it is common to perforate such bags to prevent such deterioration and to minimize high humidity in the package, which encourages microbial growth.
Shippers of fresh vegetables and vegetable processors appreciate the perishability of vegetables and do everything they can to minimize delay in processing of the fresh product. In many processing plants it is common practice to process vegetables immediately from the fields. To ensure a steady supply of top-quality produce during the harvesting period, many large food processors employ trained filed managers who can advise on growing practices so that vegetables will mature and can be harvested in rhythm with the processing plant capabilities. This minimizes pileup and need for storage.
Postharvest Practices
Cooling of harvested vegetables in the field is a common practice (see Fig. 9.2). Fresh produce is often transported in liquid-nitrogen-cooled trucks to processing plants or directly to market. At the processing plant, vegetables are cleaned, graded, peeled, cut, and so forth; some equipment is used for these operations, but use of hand labor is still common.
Washing
The choice of washing equipment and other equipment used in processing vegetables depends on the size, shape, and fragility of the particular kind of vegetable.
A flotation cleaner for peas and other small vegetables (Fig. 18.3) operates on the principle that sound peas will sink, whereas broken peas, weed seeds, and certain other kinds of contamination will float, provided a liquid of the proper density is employed. In this case, a mineral oil-water emulsion is used and its density can be further controlled by frothing the mixture with air. The sound peas that sink are moved on to further processing and the floating debris is pumped to waste. Another type of washer is the rotary washer in which vegetables are tumbled while they are sprayed with jets of water. This type of washer should not be used to clean fragile vegetables. Fragile vegetables such as asparagus are valued for their wholeness and cannot be washed in agitating equipment that would break them up. Asparagus may be washed by gentle spraying on a belt.
Vegetables are washed to remove not only field soil and surface microorganisms but also fungicides, insecticides, and other pesticides. There are laws specifying maximum levels of these contaminating materials that may be retained on vegetables. Modern instruments can detect many pesticide residues at levels as low as a few parts per billion. Wash water containing detergents and other sanitizers can reduce the level of many residues.
Figure 18.3
Skin Removal
Several methods are used to remove skins from those vegetables requiring skin removal. Skins can be softened from the underlying tissue by submerging vegetables in hot alkali solution. Lye may be used at a concentration of about 1% and at about 93C. The vegetables with loosened skins are then conveyed under high-velocity jets of water which wash away the skins and any residual lye (see Fig. 5.3). Since the cost of lye and of treating lye-containing waste waters can be appreciable, processors sometimes use less expensive hot-water scalding followed by a machine that slits the skin and gently squeezes the vegetable, such as tomatoes, through the slit skin.
Vegetables with a thick skin, such as beets and sweet potatoes, may be peeled with steam under pressure as they pass through cylindrical vessels. This softens the skin and the underlying tissue. When the pressure is suddenly released, steam under the skin expands and causes the skins to puff and crack. The skins are then washed away with jets of water.
Onions and peppers are best skinned by exposing them to direct flame or to hot gases in rotary tube flame peelers of the type shown in Fin. 18.4. Here, too, heat causes steam to develop under skins and puff them so that they can be washed away with water.
Cutting and Trimming
Many vegetables require various kinds of cutting, stemming, pitting, or coring. Asparagus spears are cut to precise length. The clippings from the base of the stalk, which are more fibrous and tougher than the prized stalk, are used in soups and other heated products where heat tenderizes them. Brussels sprouts are trimmed largely by hand by pressing the base against a rapidly rotating knife. Green beans are cut by machine into several different shapes along the length of the vegetable or transverse to the length. Olives are pitted by aligning them in small cups and then mechanically pushing plungers through the olives (Fig. 18.5). Pimentos may then be mechanically stuffed into the holes.
Figure 18.4
Blanching
Most vegetables that do not receive a high-temperature heat treatment (as in normal canning) must be heated to a minimal temperature to inactivate natural enzymes before processing or storing (even when frozen). This special heat treatment to inactivate enzymes is knowns as blanching. Blanching is not indiscriminate heating. Too little is ineffective, and too much damages vegetables by excessive cooking, especially when the fresh character of the vegetable is to be preserved by freezing. Blanching is essential for vegetables that are to be frozen because freezing only slows enzyme action, it does not destroy or completely stop it. If blanching does not precede freezing, then the product, which is often held in the frozen state for many months, will slowly develop off-flavors and off-colors, and other kinds of enzymatic spoilage may result.
Two of the more heat-resistant enzymes in vegetables are catalase and peroxidase. If these are destroyed, then other enzymes in vegetables are catalase and peroxidase, inactivated also. Effective heat treatments for destroying catalase and peroxidase in different vegetables are known, and sensitive chemical tests have been developed to detect the amounts of these enzymes that might survive blanching treatment.
Because various types of vegetables differ in size, shape, heat conductivity, and the natural levels of their enzymes, blanching treatments had to be established on an experimental basis. As with sterilization of food in cans, the larger the food item, the longer it takes for heat to reach the center. Peas are more rapidly blanched than corn on the cob. Small vegetables may be adequately blanched in boiling water in a minute or two; large vegetables may require several minutes (Table 18.4). Blanching with steam under pressure at higher temperatures requires shorter times but runs a greater risk of heat damage to the vegetable.
Figure 18.5
Because much of the enzyme activity in sweet corn is within the cob, steam blanching (Fig. 18.6) to inactivate 100% of the enzyme activity requires excessive heat. Therefore, as a compromise, processors use blanching conditions that destroy only about 90% of the enzyme activity; this avoids excessive softening of the kernels, which would reduce the quality of the final, frozen product more than the slight residual enzyme activity does. Blanching with microwave energy, to rapidly heat the center of large items before the surfaces are overcooked, can be effective in applications such as this.
Canning.
Large quantities of vegetable products are canned. A typical flow sheet for a vegetable canning operation (which also largely applies to fruits) is shown in Fig. 18.7. The unit operation performed in sequence include harvesting, receiving, washing, grading, heat blanching, peeling and coring, can filling, exhausting to remove air, sealing, retorting, cooking, labeling, and packing. The vegetable may be canned whole, diced, pureed, as juice, and so on.
HARVESTING AND PROCESSING OF FRUITS
As with vegetables, the diversity of kinds of fruit is further enlarged by the numerous cultivars of a given fruit. There are, for example, about 1000 varieties of apples and about 3000 cultivars of pears, but of these only a few are commercially important. Although some fruit is marketed fresh, in many cases more is processed into a wide range of products. Varietal differences are particularly important in selecting fruits for use in various products. Apples provide a good illustration. In addition to begin eaten fresh, apples are used to make applesauce, canned apple slices, apple juice and cider, jellies, frozen slices, and dried slices. Apple cultivars differ in such properties as resistance to weather, insects, and disease; time of maturity and yield; storage stability; color of flesh; firmness when cooked; amount of juice; acidity; and solids content. For optimum results apple cultivars must be matched to particular end uses, and processing plants frequently are equipped to manufacture the products for which the local apple cultivars are best suited. This is also true of other fruits. Knowledge of varietal differences for the various fruits is highly specific; for this reason, when a fruit processing use is contemplated, it is best to consult with state agricultural experiment stations or equivalent agencies.
Fruit Quality
Fruits quality depends on tree stocks, growing practices, and weather conditions. More important, however, are the degree of maturity and ripeness when picked and the method of harvesting. There is a distinction between maturity and ripeness of a fruit. Maturity is the condition when the fruit is ready to eat or, if picked, will become ready to eat on further ripening. Ripeness is that optimum condition when color flavor, and texture have developed to the peak. Some fruits are picked when they are mature but not yet ripe. This is especially true of very soft fruits like cherries and peaches; when fully ripe such fruits are so soft as to be damaged by the act of pickling.
Figure 18.7
Further, many fruits that continue to ripen off the tree are likely to become overripe before they can be utilized if picked at peak ripeness.
When to Pick
The proper time to pick fruit depends on several factors: the cultivar, location, weather, ease of removal from the tree which changes with time, and he purpose to which the fruit will be put. In oranges, for example, both the sugar and acid levels change as fruits ripen on the tree (sugars increase and acid decreases). The ratio of sugar to acid determines the taste and acceptability of the fruit and the juice. Since citrus fruits cease ripening once they are picked, the quality of citrus depends largely on harvesting at the proper time. In Florida, there are laws that prohibit picking citrus fruits until a sugar –acid ratio in that assures good quality has been reached. In the case of many fruits to be canned, fruits are picked before they are fully ripe in terms of eating texture since canning will further soften the fruit.
Several ripeness classes of honey dew melons are recognized: unripe but mature, ripening initiated, ripe, early senescence. Some of the changes in the fruit as it progresses through these stages of ripeness are indicated in Fig. 18.8. The unripe but mature stage is reached about 40 days after flowering, the earliest stage at which honey dews should be picked. If they are picked this early, however, gassing with the plant hormone ethylene is essential for proper subsequent ripening in fruit picked at the class 2 stage of ripeness, ethylene gassing may be beneficial ------ not essential for development of full ripeness. Beyond this stage, the natural production of ethylene by the fruit makes artificial gassing superfluous. Whether on the value of picked, the fruit subsequently passes through early senescence, when it is still edible but past its prime, and senescence, when it is no longer of edible quality.
Quality Measurements
Many quality measurements can be made before a fruit crop is picked to determine if proper maturity or degree of ripeness has developed. Color may be measured with instruments of the kind discussed in previous chapters, or by comparing color of fruit on the tree with standard picture charts. Because shapes on fruits change as they mature, length and width measurements also can serve as a guide to correct pickling time (Fig 18.9).
Texture may be measured by a compression device such as the simple type of plunger shown in Fig 18.10, which is pressed into the fruit and gives a reading as the spring contracts. Where individual unit of a harvest may vary, fruit may be separated after picking based on texture. For example, firm cranberries bounce, whereas soft, overripe, or rotten ones do not bounce as high. In a cranberry separator (Fig. 18.11), berries are given a chance to bounce over a wooden barrier: Those that make it are accepted; those that do not are automatically separate and used for less demanding purposes.
Figure 18.8, 18.9, 18.10 and 18.11
As fruits mature on the tree, their concentration of juice can be estimated with a refractometer or a hydrometer. The concentration of soluble solids in the juice can be estimated with a refractometer or a hydrometer. The former measures the ability of solutions to bend or refract a light beam, which is proportional to the solution’s concentration; a hydrometer is a weighted spindle with a graduated neck that floats in the juice at a height related to the juice density.
The acid content of fruit, as already mentioned, changes with maturity and affects flavor.
Acid concentration can be measured by a simple chemical titration on the fruit juice. But for many fruits the tartness and flavor are determined primarily by the ratio of sugar to acid. Percentage of soluble solids, which are largely sugars, is generally expressed in degrees Brix, which relates specific gravity of a solution to an equivalent concentration of pure sucrose. Therefore, in describing the taste or tartness of fruits and fruit juices, the terms sugar-to-acid ratio or Brix-to-acid ratio are commonly used. The higher the Brix, the greater the sugar concentration in the juice; the higher the Brix-to-acid ratio, the sweeter and less tart is the juice. The seasonal changes in degrees Brix, and acid content, the Brix-to-acid ratio for grapefruit juice are summarized in Table 18.6.
Harvesting and Processing
Much of the harvesting of the most fruits crops is still done by hand. This labor may represent about held of the cost of growing the fruit. Therefore, development of mechanical harvesters remains a top priority for agricultural engineers; also important is associated research to breed varieties that produce fruits of nearly equal size that mature uniformly and are resistant to mechanical damage. Mechanical damage can be subtle. For example, cherries that are not to be processed immediately frequently are picked with their stems attached to avoid the small break in the flesh that would allow microbial invasion. This is why fresh cherries with stems are sometimes seen in the supermarket.
A- These standards as established by the USDA are subject to frequent revision.
B- When the Brix is above 16, the ratio may be less than 10:1
C -When the Brix is above 16. The ratio may be less than 9:1.
Harvested fruit is washed to remove soil, microorganisms, and pesticide residues and then sorted according to size and quality. Sorting techniques have progressed from hand sorting to water shorting, which takes advantages of changes in density with ripening, to sophisticated automatic high-speed sorting in which compressed air jets separate fruit in response to differences in color and ripeness as measured by light reflectance or transmittance. Fresh fruit is not marketed as such may be processed in many ways, one of the more important being freezing.
Freezing
Large amounts of high-quality fruit are frozen for home, restaurant, and manufacturing use by the baking and other food industries. Freezing is generally superior to canning for preserving the firmness of fruits. As with vegetables, fruit to be frozen must be stabilized against enzymatic changes during frozen storage and on thawing.
The principal enzymatic changes that are objectionable in the case of frozen fruits are oxidation, which cause darkening of color and alterations of flavor. A particularly important color change is enzymatic browning of lighter colored fruits such as apples, peaches, and bananas. This is due to oxidation of pigment precursors, often referred to as catechol-tannin substrates, by enzymes of the group known a phenol oxidases and polyphenol oxidases. Depending on the intended end use for the frozen fruit, various methods are employed to inactivate these enzymes or otherwise prevent oxidation.
Heat Blanching. Fruits generally are not heat-blanched because the heat causes loss of turgor, resulting in sogginess and juice drainage after thawing. Instead, chemicals are commonly used without heat to inactivate oxidative enzymes or to act as antioxidants; these chemicals are combined with other treatments as described in the following sections. An exception exists in the case of fruit slices to be frozen for later use in pies. Since the frozen fruit ultimately will receive heating during the baking operation, heat blanching before freezing is still sometimes practiced. In this case, calcium salts may be added to the blanching water, or added after blanching, to firm the fruit by forming calcium pectates. It also is not uncommon –to add pectin, carboxymethyl cellulose, alginates and other colloidal thickeners to such fruit prior to freezing.
Ascorbic Acid Dip. Ascorbic acid or vitamin C minimizes fruit oxidation primarily by acting as an antioxidant and itself becoming oxidized in preference to the catechol tannin compounds. Ascorbic acid frequently is added to fruits dissolved in a sugar syrup. Levels of 0.05-0.2% ascorbic acid in an apple-syrup or peach-syrup mixture usually are effective, provided there is time of penetration prior to the freezing step. Peaches so treated may not darken in frozen storage at -18C in 2 years. Because increased acidity also helps retard oxidative color changes, ascorbic acid and citric acid may be used together. Citric acid further reacts with (chelates) metal ions and, ------------------
Sulfur Dioxide. Sulfur dioxide (SO2) is able to stabilize the color of fresh and processed fruits and vegetables. Sulfur dioxide also inhibits the activity of common oxidizing enzymes and has antioxidant properties; that is, it is an oxygen acceptor as is ascorbic acid). Further, SO2 reduces nonenzymatic Maillard-type browning by reacting with aldehyde groups of sugars so that they are no longer free to combine with amino acids. Sulfur dioxide also interferes with microbial growth. Inhibition of browning is especially important in dried fruits such as apples, apricots, and pears.
Sulfur dioxide dips have been used to prolong the fresh appearance of cut vegetables and lettuce and other uncooked vegetables in restaurants and salad bars. However, some people are severely allergic to SO2; thus, the FDA has prohibited the use of SO2 on fresh produce and has required that uses in processed products be limited to a residual of less than 10 parts per million or appropriately labeled so that sensitive individuals can avoid exposure.
Sugar Syrup. The addition of sugar syrup, one of the oldest methods of minimizing oxidation, was used long before the browning reactions were understood and remains today a common practice. Sugar syrup minimizes oxidation by coating the fruit and thereby preventing contact with atmospheric oxygen. Sugar syrup also offers some protection against loss of volatile fruit esters, and it contributes sweet taste to otherwise tart fruit. Today it is common to dissolve ascorbic acid and citric acid in the sugar syrup for added effect or to include sugar syrup after an SO2 treatment.
Vacuum Treatment.
When employed, vacuum treatments generally are used in combination with one of the chemical dips or with the addition of sugar syrup. The fruit submerged in the dip or in the syrup is placed in a closed vessel and vacuum is applied to draw air from the fruit tissue. When the vacuum is broken, the chemical dip or syrup enters the voids from which air was removed, effecting better penetration of the solution.
Concentration and Drying.
Some high-moisture fruits may be purred and concentrated to two or three times their natural solids content for more economical handling and shipping, or the fruits may be dried for various purposes to different moisture levels. Partially dried fruits (e.g., dried apricots, pears, prunes, figs, and raisins) are still largely prepared by sun drying in open wooden trays. When fruits are dried under temperature conditions that do not inactivate oxidative enzymes, SO2 is commonly employed to minimize browning, as mentioned earlier. The SO2 also keeps down microbial growth during the slow, low-temperature drying process.
FRUITS JUICES
Perhaps the most commonly processed juice is orange juice. However, several of the steps used in orange juice also are common in the manufacture of other juices, but equipment varies depending on the properties of the different fruits. The main steps in the production of most types of juice are extraction of the juice, clarification of the juice, juice deaeration, pasteurization, concentration (if solids are to be increased), essence add-back, canning or bottling, and freezing if the juice is to be marketed in this form.
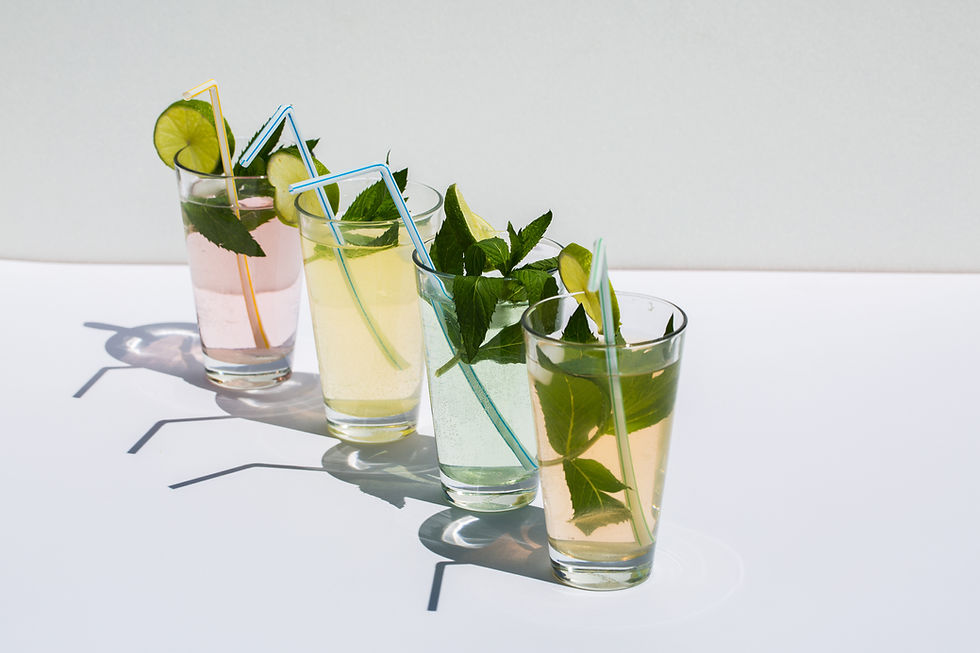
Extraction
Juice extractors for oranges and grapefruit, whose peels contain bitter oils, are designed to cause the peel oil to run down the outside of the fruit and not enter the juice stream. Because bitter peel oil is not a problem in the case of apples, the whole apple is pressed after grinding.
Clarification
The juice pressed from most fruits contains small quantities of suspended pulp, which is often removed. This may be done with fine filters, but since these have a tendency to clog, it is common to use high-speed centrifuges, which separate the juice from the pulp according to their differences in density.
Many people prefer crystal clear apple juice. However, simple filtration of centrifugation may leave minute particles of pulp and colloidal materials suspended in the juice by the natural pectic substance of the fruit. Addition of commercial enzyme preparations that digest pectic substances causes the fine pulp to settle, which makes filtering or centrifuging more effective and produces clarified apple juice. Orange juice, on the other hand, is more acceptable if it retains a slight cloud of suspended pulp and so this is not removed.
Deaeration
orange and other juices contain entrapped air and are deaerated by being sprayed into a vacuum deaerator (Fig. 18.12). This minimizes subsequent destruction of vitamin C and other changes due to oxygen.
Additional Steps
Generally, fruit juices are pasteurized to decrease microbial growth and to inactivate natural enzymes. All natural juices are low in solids, and so it is common to concentrate many of them whether they are to be frozen or not. When this is done, low-temperature vacuum evaporation generally is employed to retain maximum flavor. Nevertheless, removal of water is always accompanied by the evaporation of some of the juices volatile essences. Therefore, the evaporated water and essence coming from the vacuum evaporator is not discarded but is passed through and essence-recovery unit. Such units distill the essence from the water and re-condense it. The essence is then added back to the concentrated juice to enhance flavor. Various methods of concentrating juices, including pumping them through reverse-osmosis membranes (Fig. 18.13), are currently being studied. Reverse osmosis can be less costly than vacuum evaporation; further, special membranes can retain the essence in the juice concentrate since many essence molecules are too large to pass through them. Membrane concentration requires dioxide, and acidity for various beverages are given in Table 19.2, although the products of different manufacturers may vary somewhat from these values.
Figure 18.12
That pulp first be centrifuged from the juice to prevent membrane clogging. Pulp can then be added back to the concentrated juice. The juice concentrate may then be frozen, or it may be shipped for subsequent reconstitution and packaging as single-strength juice.
In recent years it has become popular to blend different juice types together to form new beverages. Mango may be blended with apple and cranberry or citrus juices with raspberry. Pear juice is a popular base for many juices because it has a strong fruit flavor but is not highly characteristics of any one fruit.
Many juices and juice blends are rich in vitamin C. Apple juice, which normally is low in vitamin C, may be fortified with this vitamin. There is increasing demand for high quality juice as a nutritious beverage and much is now being aseptically packaged in paper cartons of individual serving size.
Whenever fruit is processed or juice produced, there remain peels, pits, and other non-juice solids. Some of this finds its way into confectionery and jelly products, pectin manufacture, recovery of chemicals, and animal feeds.
Figure 18.13
BIOTECHNOLOGY
For many decades, plants have been bred or mutated in order to produce offspring which have desirable characteristics such as disease and insect resistance or eating and processing qualities. This is done by selective breeding or direct mutation, both of which alter the genetic makeup of the plant by altering its DNA. It is this breeding that gives us so many types of apples, for example.
In recent years, scientists have learned how to more directly influence the genetics of plants by inserting specific pieces of DNA into a plant’s DNA. Thus, inheritable and specific properties or traits can be incorporated into the genetics of plants. Desirable traits such as resistance to plant diseases and viruses or quality and storage attributes can be specifically “engineered” into plants.
A couple of examples illustrate the usefulness of such techniques. Squash, like other plants, are commonly infected by a pathogenic virus that causes decreased yields and quality. By incorporating a portion of the DNA from the virus into the squash’s DNA, the plant acquires resistance to the virus. Another example comes from a bacterium which is pathogenic to certain insects. This bacterium is known as “Bacillus thuringiensis” and produced a protein which is quite toxic to insects but harmless to mammals. Insertion of the DNA which tells the bacterium to make this protein into a plant gives the plant the ability to form the protein as a normal part of growing. When the protein is produced by the plant, insects consume the plant, they also consume some of the toxic protein. Thus, insects can be controlled without the application of pesticides. It can be expected that the use of biotechnology to improve crops will accelerate in the next decade. This will likely result in increased crop yields and improved quality.
Comments